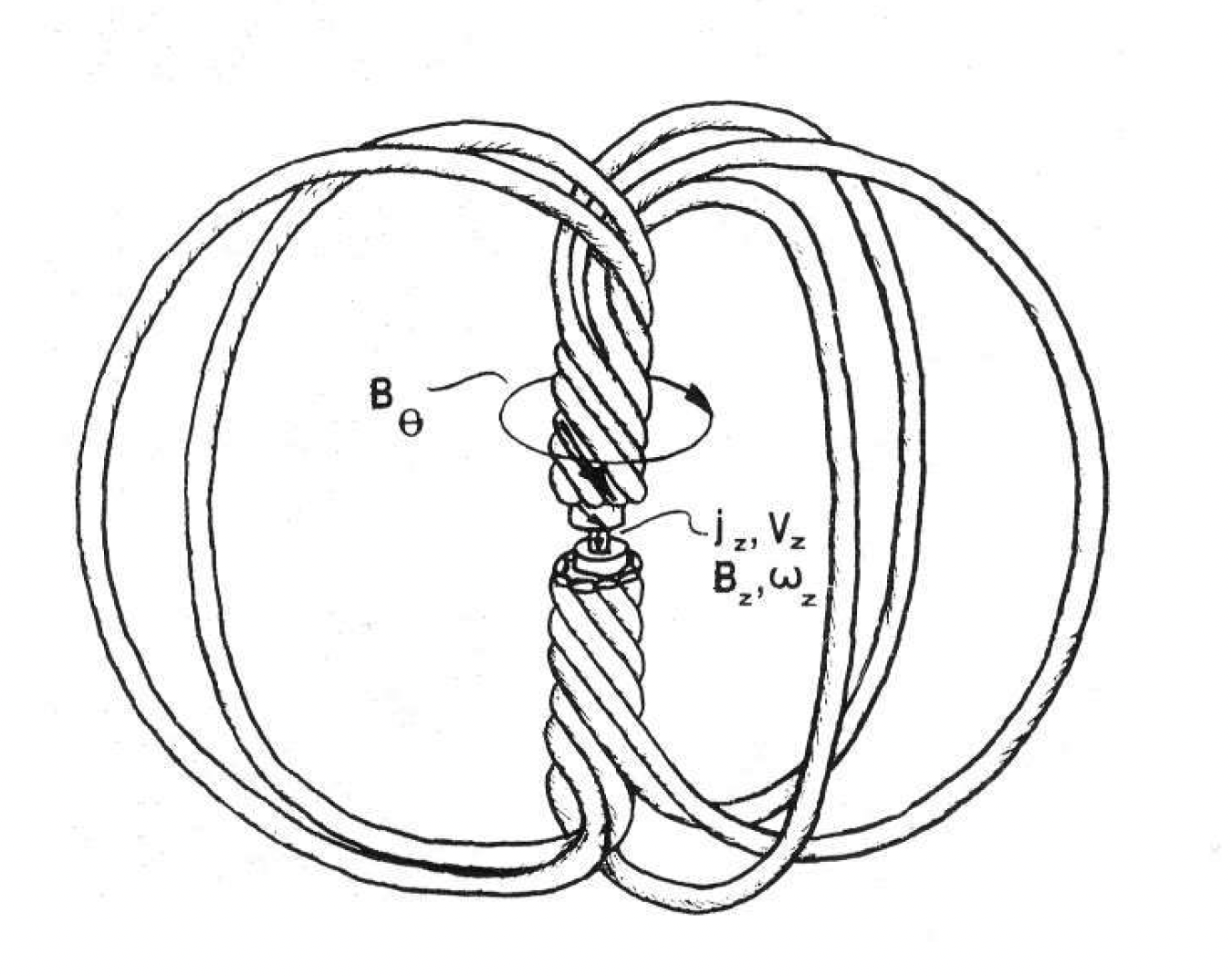
Nice but that question is likely too early. I do think the issue is boundedness which a neo crystalline structure maintains and that is my approach with Cloud cosmology. Trying something else is likely to end up with all sorts of things like the above image.
It may be helpful or not and just how do we confirm?
So I am neutral at best here.
Summary:Is the Proton a Plasmoid? (Neutron, too?)
Imitating Nature: The Fastest Route to Fusion
Is the Proton a Plasmoid?
(Neutron, too?)
https://mailchi.mp/lppfusion/part-2-of-march-16-2023-report-8754157?e=3eee1c4ccd
Plasma structures that are confined by their own magnetic fields, called plasmoids, are central to LPPFusion’s approach to fusion energy generation. It is in the dense, hot plasmoids that the fusion reactions in our FF-2B device take place. Researchers have long known that plasmoids are formed in nature at much larger astrophysical scales in the sun’s atmosphere, in the formation of stars, all the way up to giant quasars. We’ve used observation of astrophysical objects to create our theories of plasmoids and filament in our dense plasma focus device (DPF). We imitate nature as the fastest path to fusion.
As we prepare for experiment this year with pB11 fuel, we know that important things will be quite different for our micron-sized plasmoids than for giant ones in solar flares. For one thing, quantum effects will be much more important.
But now, exciting new experiments at much smaller scales , probing the structure of neutrons and protons, have raised the possibility that plasmoid-like objects may exist at those scales as well. Indeed, it’s even possible, although far from established, that plasmoids may be central to the nature of the nuclear fusion energy that drives the sun, the stars, and the entire universe.
These new discoveries will help us at LPPFusion to scale UP predictions from the very small, where quantum effects dominate, as well as from the very large. We think this will speed our work with hydrogen-boron ( pB11) fusion fuel in the coming year, and open up new opportunities for collaboration with other researchers at the cutting edge of basic and applied research.
The new experimental observations, published in November, 2022 in Nature, joined related surprising results from 2021 in deepening a 35-year-old mystery about the internal structure of the proton. Protons, neutrons and electrons are the basic constituents of all matter. Scientists have known from observations since the 1930’s that protons have some sort of internal structure, but have long struggled to understand what that structure is. The widely-accepted current model, first formulated in 1964, describes protons as each made up of three “quarks”—hypothetical point-particles that only exist inside protons. While popular in the field, the model has not been able to predict key properties of protons, especially their ability, together with neutrons, to hold the nuclei of atoms together through the nuclear force. It is this nuclear force that gives rise to fusion reactions and fusion energy.
Our actual, practical, understanding of nuclear forces and nuclear reactions comes not from any theory of the structure of the proton or neutron, but from many thousands of careful experimental measurements of how fast the reactions actually occur in various conditions. So, the lack of a good theory of the proton structure has not visibly held the field back.
Yet the history of physics shows that each time a good model of the structure of matter has been achieved, far-ranging practical results follow. This was the case, for example for the quantum theory of the structure of the atom, which is now the basis for much of modern technology. The decades-long search for the structure of the proton thus has good scientific motivation.
Strong evidence against the quark theory first emerged back in 1987 when Dr. Alan Krisch and colleagues at the University of Michigan demonstrated in accelerator experiments that protons have a far greater chance of being deflected in a collision when the spins (axes of rotation) are aligned and that they are three times more frequently deflected in the direction of the spins, rather than opposite to the spins. They acted like tiny vortices pushing each other about. A Krisch pointed out, this could not possibly happen if protons were just bags of three quarks and contradicted the clear predictions of the quark theory. No answer to the “proton spin problem” emerged in subsequent decades.
Instead, high-energy and particle physicists continued their program of smashing protons (and electrons) together at higher and higher energies to try to probe their structure, a project often compared, by advocates and critics alike, to “colliding two Swiss watches together” and trying to make sense of the pieces.
In the past few years, groups of researchers have taken a different tack that may lead to unraveling the mystery. They’ve been probing protons and neutrons with relatively low-energy electrons, using a tweezers instead of a hammer on the nuclear watch-works.
In this way they could find the distribution of spin, or angular momentum, within both the proton and neutron. What they found and published in 2021 was a big surprise to them and in complete contradiction to the quark model. While the model predicted that spin, like other properties, would be simply concentrated toward the centers of proton and neutron, the measurement showed that spin was instead concentrated in a hollow shell or ring structure. (Fig.1) This shell structure of spin makes sense of the strong spin effect found by Krisch, just as a long baseball bat conveys angular momentum far more efficiently than a short barbell.
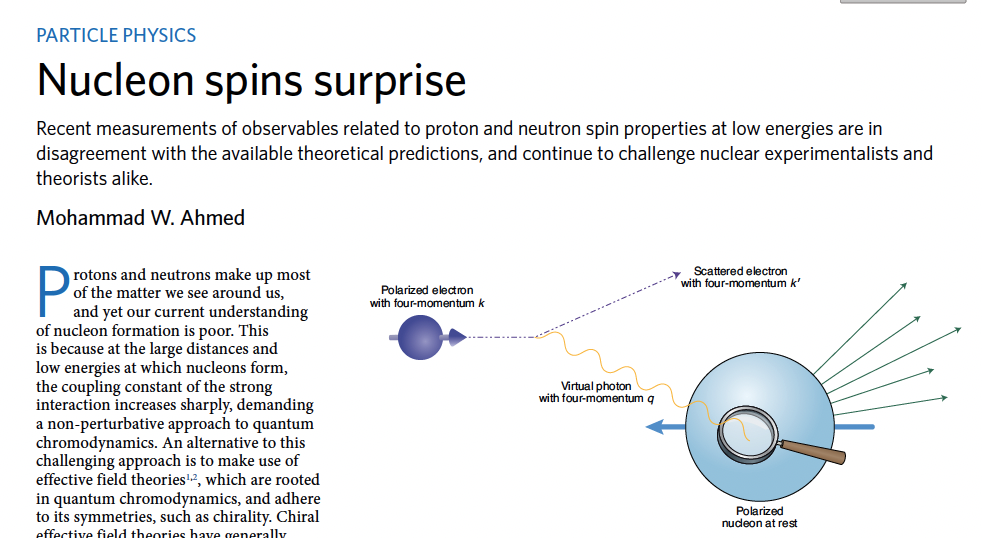
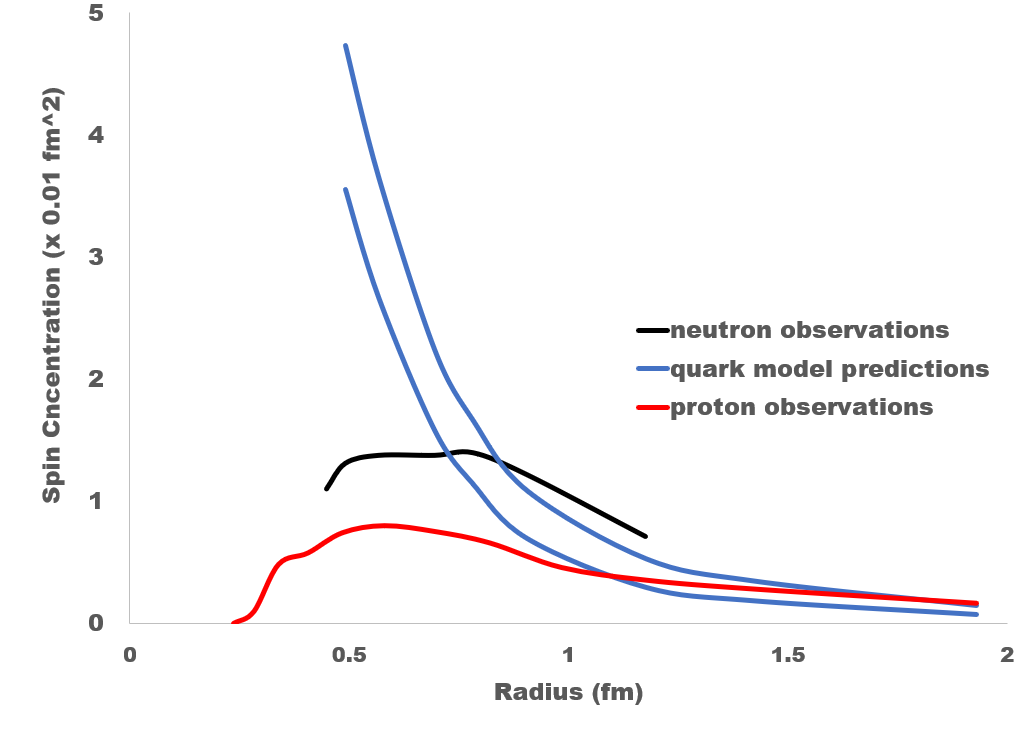
Fig. 1 June 2021 experiments used electrons to probe the spins of protons and neutrons and got results that greatly surprised researchers (top image from Nature Physics). While the quark model predicted the spins would be sharply concentrated towards the center of the particle (blue lines) observations of neutrons(black) and protons(red) showed a ring or shell of spin. (data from experiments replotted by LPPFusion)
The new Nature paper both deepened the contradiction with the quark model and provided important clues to an alternative one. These new experiments probed the electric and magnetic fields within the proton indirectly by measuring quantities called “electric polarizability” and “magnetic polarizability”. These quantities basically measure how “soft” a charged, magnetized object is to being deformed by external fields and the directions of the deformations. Generally, regions of stronger fields inside an object correspond to stiffer regions.
Again, the quark model simply predicted stiffness would increase smoothly toward the center of the proton. Instead, the research team found that there was a surprising shell of softness inside a stiffer shell. The 0.6 fm (femtometer) radius of the stiffer zone corresponded to that of the spin shell found in 2021. ( For comparison the outer radius of the proton is around 1 fm). A second stiffer, high field, region was found at a radius of 0.25 fm. But most strikingly, the direction of the deformation and, probably, of the internal fields, switched directions twice at the stiffest, highest-field radii (Fig. 2).
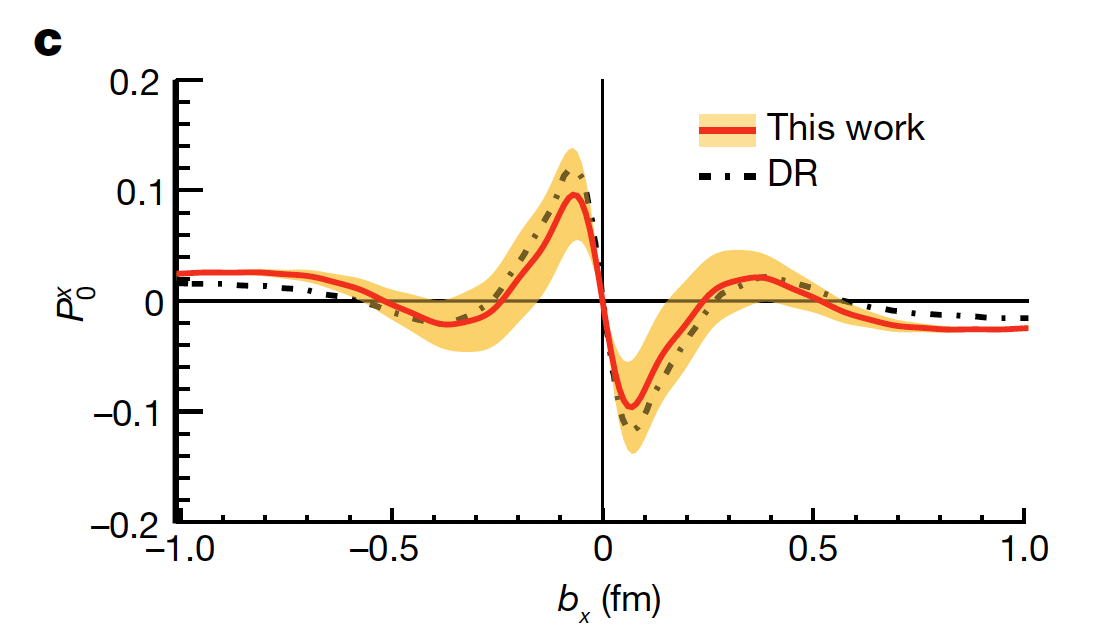
Figure 2. In the new results published Nov. 2022, the direction of the deformation of the electromagnetic field in the proton changes twice as the radius probed a shell at a radius of 0.6 fm. This profile is consistent with that observed in plasmoids observed at much large scales.
Protons as Plasmoids
What is particularly exciting about the new results is that they are what would be expected if the magnetic fields within the proton were produced by a tiny plasmoid. Plasmoids, plasma structures that are self-contained by magnetic fields produced by currents within the structures, were first discovered experimentally and named in 1957 by Winston Bostick at Stevens Institute in NJ. Following the invention by others of the dense plasma focus (DPF) device in 1964, Bostick and his close collaborator Vittorio Nardi, also at Stevens, became pioneers in the development of this fusion device.
By 1975, Bostick and Nardi had discovered that the fusion reactions in the DPF were produced in a tiny plasmoids that had a very specific structure, made up of force-free filaments of current, twisted into a spherical vortex. (Fig. 3). At this time, Bostick informed Eric Lerner, now LPPFusion Chief Scientist, about the DPF. Starting in 1981, with help from Nardi, Lerner used the DPF as a model for plasmoids on an astrophysical scale, especially quasars. This work became the basis for a more elaborated versions of the Bostick-Nardi theory of the DPF—and the basis of LPPFusion’s present development of Focus Fusion.
Bostick, in 1985, turned to the very small and proposed the filamentary plasmoid as a model for the electron. Bostick’s initial model was not an exact copy of the plasmoids actually observed, as he decided to add a gravitational force term to his model. This was not at all observed in the DPF, where gravitational forces are negligible compared with electromagnetic ones. This additional concept turned out to be an error (even the best researchers make them!).
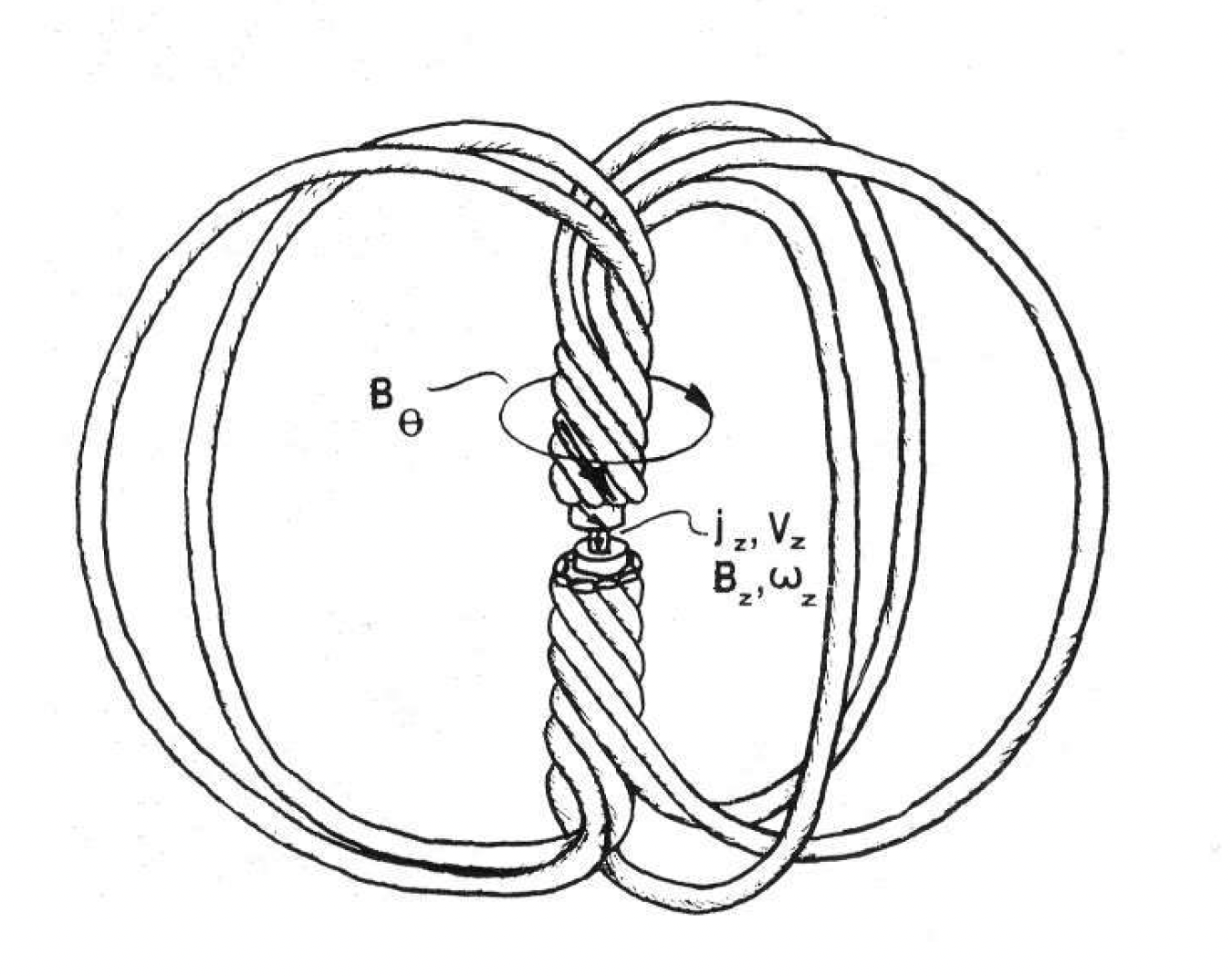
Figure 3. The structure of plasmoids discovered by Bostic and Nardi in 1975 is illustrated in this drawing from one of their key papers. Filaments of current and magnetic field (with the currents running along the field lines) are twisted into a tight core and then circulate back in a shell at greater radius. In LPPFusion’s FF-2B experimental fusion device, plasmoids like this produce fusion reactions in the dense core. This structure fits data newly found for protons and neutrons.
However, several researchers inspired by Bostick’s work, and independently of it, did in subsequent years create models of nucleons that were wholly electromagnetic in nature, imitating in various ways the experimentally-observed plasmoids. In these models, the attractive nuclear force is actually the attractive force of magnetic fields in the filaments of the plasmoids.
The new results reported in Nature, together with the 2021 observations make these magnetic models far more likely to be valid. As anyone who has played with magnets knows, magnetic forces fall off very quickly with distance. In the standard conception of nucleons, with electric and magnetic fields all concentrated towards the center, the magnetic forces between protons and neutrons would be far too weak to power nuclear attraction. But the new results show that in reality the fields are concentrated towards the outer surface of the protons and neutrons. There, the fields of the two particles can come much closer to each other, providing the right amount of force and energy to explain nuclear attraction—and fusion reactions.
Indeed, the new results are compatible with a model of neutrons and protons binding together as two counter-spinning plasmoids with their filaments meshing as they spin like the gears in a very tiny Swiss watch.(Fig.4)
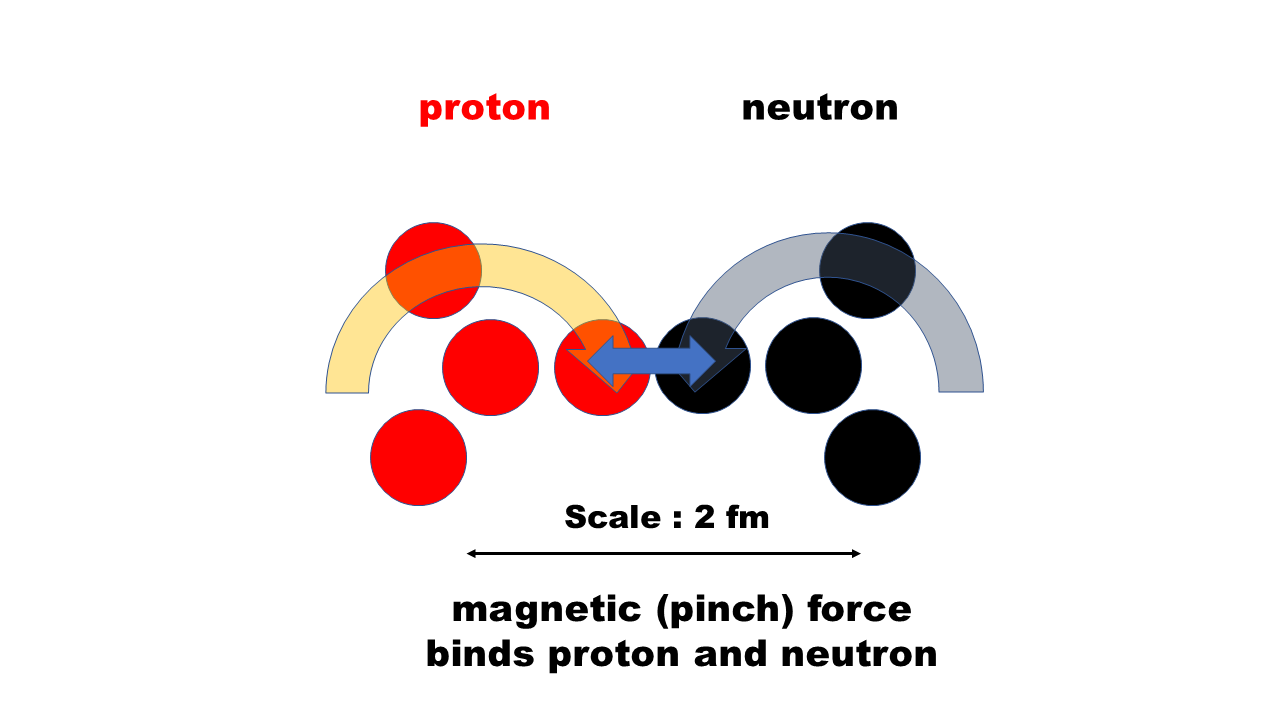
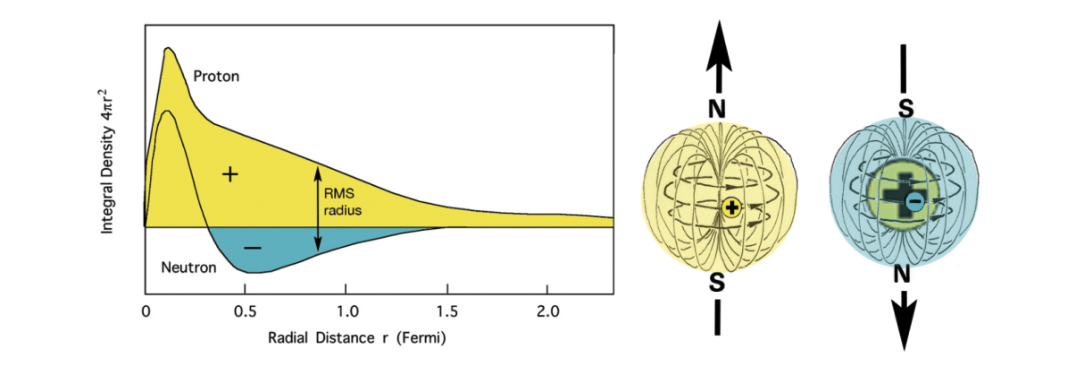
Figure 4. The new data on neutrons and protons is compatible with a model of nuclear forces that are wholly magnetic in nature and are generated by plasmoids. For example, a deuteron, the nucleus of deuterium, binding one proton (top, red) with one neutron (top, black) is shown in “top-down” cross section in this image by LPPFusion Chief Scientist Eric Lerner. In each particle, a ring of three filaments circulates together through a central core, as in Fig. 3. As the two particle spin in opposite directions, the filaments bind to each other in turn through magnetic force, creating the strong nuclear attraction (blue arrow between proton and neutron filaments, top center).From the side the plasmoids will look something like the image at lower right (from a 2013 paper hypothesizing the magnetic model of nuclear forces by V. Dallacasa and N. D. Cook). While electric currents in the neutral neutron may seem strange, scientist have long know that the neutron has a positively charged core surrounded by a negatively charged halo (graph lower left, from same 2013 paper).
We emphasize that these exciting new results are far from actually demonstrating that protons and neutrons are plasmoids, although they are evidence towards that conclusion. To really validate such models, researches have to mathematically elaborate them to make concrete new predictions that can be tested by further experiments.
This is where we believe new collaborations will be possible that will speed our work to pB11 fusion. LPPFusion Simulation Research Dr. Warwick Dumas has been working to develop a simulation of the DPF plasmoid to help guide our coming experiments. These simulations will have to include the important quantum magnetic field effects that help keep the plasma hot. Not surprisingly, adding quantum effects to electromagnetic equations does not make them easier to solve or simulate.
But with the new discoveries we believe other researchers will be eager to collaborate with us on simulations that cover the range of scales from our micron-sizes all the way down to the proton’s. We think this will open up, over the coming year or so, rapid advances linking basic nuclear and applied fusion research, advances which may uncover other quantum effects that can boost our plasmoids to net energy by the fastest path possible—by imitating what occurs in nature, from the nucleus to the cosmos.
No comments:
Post a Comment