This chapter is very welcome as most of us have an intuitive understanding of plasma and expect little more when it comes to mathematical modeling. This item bears this out wonderfully as our best foundational concept just happens to be a three dimensional fractal allowing for disturbances or instabilities as well.
We can add one other critical aspect to all our attempts to understand the nature of the Space Environment. The physical reality of the second tier as a non compressible fluid reacting weakly and naturally neutralizing free electrons. This is all possible because all components at the electron scale react along an axis rather that through a spherical continuous field as we naturally observe in the third tier environment.
What this means is that free electrons are available everywhere to be tapped by spiral fields reacting anywhere. This constantly release powerful potentials and just as surely locks them back down. Except that they moved in the process without encountering resistance.
The interesting speculation is whether boundedness exists at the Galactic scale. It appears plausible.
.
Essential Guide to the EU – Chapter 3 Plasma
3.1 Introducing Plasma
https://www.thunderbolts.info/wp/2011/10/25/essential-guide-to-the-eu-chapter-3/
It is known that space is filled with
plasma. In fact, plasma is the most common type of matter in the
universe. It is found in a wide range of places from fire, neon lights,
and lightning on Earth to galactic and intergalactic space. The only
reason that we are not more accustomed to plasma is that mankind lives
in a thin biosphere largely made up of solids, liquids, and gases to
which our senses are tuned. For example, we don’t experience fire as a
plasma; we see a bright flame and feel heat. Only scientific experiments
can show us that plasma is actually present in the flame.
While plasma studies may focus on a single subject such as fusion energy production, the understanding of how the Universe operates also awaits the student with a wider interest.
Image credit: DOE-Princeton Plasma Physics Lab; Peter Ginter
“Plasma is a collection of charged particles that responds collectively to electromagnetic forces” (from the first paragraph in Physics of the Plasma Universe,
Anthony Peratt, Springer-Verlag, 1992). A plasma region may also
contain a proportion of neutral atoms and molecules, as well as both
charged and neutral impurities such as dust, grains and larger bodies
from small rocky bodies to large planets and, of course, stars.
The defining characteristic is the
presence of the free charges, that is, the ions and electrons and any
charged dust particles. Their strong response to electromagnetic fields
causes behavior of the plasma which is very different to the behavior of
an un-ionized gas. Of course, all particles – charged and neutral –
respond to a gravity field, in proportion to its local intensity. As
most of the Universe consists of plasma, locations where gravitational
force dominates that of electromagnetism are relatively sparse.
Because of its unique properties, plasma
is usually considered to be a phase of matter distinct from solids,
liquids, and gases. It is often called the “fourth state of matter”
although, as its state is universally the most common, it could be
thought of as the “first” state of matter.
The chart below is commonly used to indicate how states change from a thermal
point of view. The higher the temperature, the higher up the energy
ladder with transitions upward and downward as indicated. However, it
takes a very high thermal energy to ionize matter. There are other means
as well, and an ionized state with charge imbalance can be induced and
maintained at almost any temperature.
A solid such as a metal electrical
cable, once it is connected in an electrical circuit with a sufficiently
high electrical voltage source (battery; powerplant) will have its
electrons separated from the metal nuclei, to be moved freely along the
wire as a current of charged particles.
A beaker of water with a bit of metallic
salt, such as sodium chloride, is readily ionized. If an electric
voltage is applied via a positive and a negative wire, the hydrogen and
oxygen atoms can be driven to the oppositely charged wires and evolve as
the gaseous atoms they are at room temperature. Such stable, neutral
states are a part of an electric universe, but this Guide will focus
more on investigating the state of plasma and electric currents at
larger scales, in space.
A molecular cloud of very cold gas and
dust can be ionized by nearby radiating stars or cosmic rays, with the
resulting ions and electrons taking on organized plasma characteristics,
able to maintain charge and double layers creating charge separation
and electrical fields with very large voltage differentials. Such plasma
will accelerate charges and conduct them better than metals. Plasma
currents can result in sheets and filamentary forms, two of the many
morphologies by which the presence of plasma can be identified.
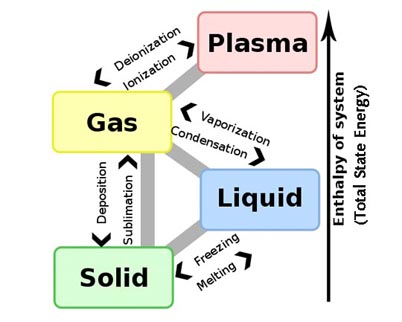
Four states or phases of matter , and the transitions between them. Note the similarity to the early Greek “primary elements” of Earth, water, air and fire. It is clear that plasma is the state with the highest energy content. Open question: From where in space does this energy come?
Image credit: Wikimedia Commons
The proportion of ions is quantified by the degree of ionization. The degree of ionization of a plasma can vary from less than 0.01% up to 100%, but plasma behavior will occur across this entire range due to the presence of the charged particles and the charge separation typical of plasma behavior.
Plasma is sometimes referred to merely
as an “ionized gas”. While technically correct, this terminology is
incomplete and outdated. It is used to disguise the fact that plasma
seldom behaves like a gas at all. In space it does not simply diffuse,
but organizes itself into complex forms, and will not respond
significantly to gravity unless local electromagnetic forces are much
weaker than local gravity. Plasma is not matter in a gas state; it is
matter in a plasma state.
The Sun’s ejection of huge masses of
“ionized gas” (plasma) as prominences and coronal mass ejections against
its own powerful gravity serves to illustrate this succinctly. The
solar ‘wind’ is plasma, and consists of moving charged particles, also
known as electric current. It is not a fluid, or a ‘wind’, or a ‘hot
gas’, to put it in plain terms. Use of other words from fluid dynamics
serves to obfuscate the reality of electric currents and plasma
phenomena more powerful than gravity, around us in space, as far away as
we can observe.
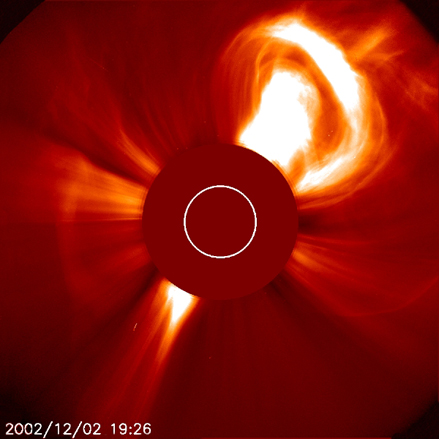
A coronal mass ejection discharges billions of tons of plasma into the interplanetary medium. The Sun is the size of the white circle on the occulting disk Courtesy, SOHO public imagery
3.2 Ionization
We know that space is filled with
fields, a variety of particles, many of which are charged, and
collections of particles in size from atoms to planets to stars and
galaxies. Neutral particles — that is, atoms and molecules having the
same number of protons as electrons, and neglecting anti-matter in this
discussion — can be formed from oppositely charged particles.
Conversely, charged particles may be formed from atoms and molecules by a
process known as ionization.
If an electron – one negative charge
– is separated from an atom, then the remaining part of the atom is left
with a positive charge. The separated electron and the remainder of the
atom become free of each other. This process is called ionization.
The positively charged remainder of the atom is called an ion. The
simplest atom, hydrogen, consists of one proton (its nucleus) and one
electron. If hydrogen is ionized, then the result is one free electron
and one free proton. A single proton is the simplest type of ion.
If an atom heavier than hydrogen is
ionized, then it can lose one or more electrons. The positive charge on
the ion will be equal to the number of electrons that have been lost.
Ionization can also occur with molecules. It can also arise from adding
an electron to a neutral atom or molecule, resulting in a negative ion.
Dust particles in space are often charged, and the study of the physics
of dusty plasmas is a subject of research in many universities today.
Energy is required to separate atoms into electrons and ions — see the
chart below.
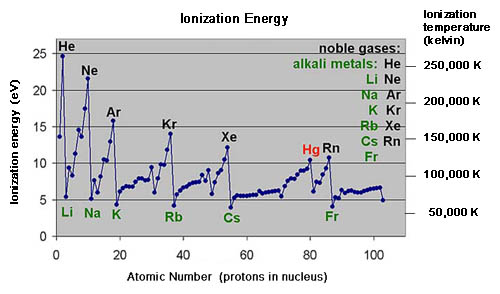
First ionization energy versus elements’ atomic numbers. Image credit:
Wikimedia Commons, edited to add temperatures along the right axis
Notice the repetitive pattern
of the chart: an alkali metal has a relatively low ionization energy or
temperature (easy to ionize). As you move to the right, increasing the
atomic number – the number of protons in the nucleus of the atom – the
energy required to ionize each ‘heavier’ atom increases. It peaks at the
next “noble gas” atom, followed by a drop at the next higher atomic
number, which will be a metal again. Then the pattern repeats.
It is interesting to note that hydrogen,
the lightest element, is considered a ‘metal’ in this electric and
chemical context, because it has a single electron which it readily
“gives up” in its outer (and only) electron orbital. Common terminology
in astronomy, in the context of the component elements in stars, is that
hydrogen and helium are the ‘gases’ and all the other elements present
are collectively termed ‘metals’.
3.3 Initiating and Maintaining Ionization
The energy to initiate and maintain
ionization can be kinetic energy from collisions between energetic
particles (sufficiently high temperature), or from sufficiently intense
radiation. Average random kinetic energy of particles is routinely
expressed as temperature, and in some very high velocity applications as
electron-volts (eV). To convert temperature in kelvins (K) to eV,
divide K by 11604.5. Conversely, multiply a value in eV by that number
to get the thermal equivalent temperature in K.
The chart above represents the ionization energy required to strip the first, outermost electron
from an atom or molecule. Subsequent electrons are more tightly bound
to the nucleus and their ionization requires even higher energies.
Several levels of electrons may be stripped from atoms in extremely
energetic environments like those found in and near stars and galactic
jets. Importance: These energetic plasmas are
important sources of electrons and ions which can be accelerated to
extremely high velocities, sources of cosmic rays and synchrotron
radiation at many wavelengths. Cosmic ray links to cloud cover patterns
affecting our global climate are reported in Henrik Svensmark’s book, The Chilling Stars.
Temperature is a measure of how much
random kinetic energy the particles have, which is related to the rate
of particle collisions and how fast they are moving. The temperature
affects the degree of plasma ionization. Electric fields aligned
(parallel) with local magnetic fields (“force-free” condition) can form
in plasma. Particles accelerated in field-aligned conditions tend to
move in parallel, not randomly, and consequently undergo
relatively few collisions. The conversion of particle trajectories from
random to parallel is called “dethermalization”. They are said to have a
lower “temperature” as a result. Analogy: think of the
vehicular motion in a “destruction derby” as “hot”, collision-prone
random traffic, and freeway vehicular movement in lanes as “cool”,
low-collision, parallel aligned traffic.
In a collision between an electron and
an atom, ionization will occur if the energy of the electron (the
electron temperature) is greater than the ionization energy of the atom.
Equally, if an electron collides with an ion, it will not recombine if
the electron has enough energy. One can visualize this as the electron’s
having a velocity greater than the escape velocity of the ion, so it is
not captured in an orbit around the ion.
Electron temperatures in space plasmas
can be in the range of hundreds to millions of kelvins. Plasmas can
therefore be effective at maintaining their ionized state. A
charge-separated state is normal in space plasmas.
Other sources of ionization energy
include high-energy cosmic rays arriving from other regions, high-energy
or “ionizing” radiation such as intense ultraviolet light incident upon
gas or weakly ionized plasma from nearby stars, an encounter between a
plasma region and a neutral gas region in which the relative velocity of
the encounter exceeds the Critical Ionization Velocity (CIV) (Hannes
Alfvén, Collision between a nonionized gas and a magnetized plasma, Rev. Mod. Phys., vol. 32, p. 710, 1960) or energetic radiative processes created within the plasma itself.
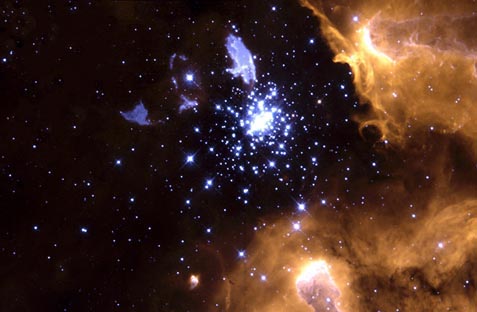
Highly energetic processes are observed in nebula NGC 3603: blue supergiant Sher 25 with toroidal ring and bipolar jets, upper center; arc and glow mode plasma discharges as emission nebula (yellow-white areas); clustered hot blue Wolf-Rayet and young O-type stars, with electric filaments and sheets throughout the dusty plasma regions of the nebula.
Image credit: W. Brandner (JPL/IPAC), E. Grebel (U. of Washington), You-Hua Chou (U. of Illinois, Urbana-Champaign), and NASA Hubble Space Telescope
In Big Bang cosmology, it is thought
that there is not enough energy in the Universe to have created and
maintained significant numbers of “loose” ions and electrons through
ionization, and therefore they cannot exist. On the other hand, whenever
ions and electrons combine into atoms, energy is given off. In the Big
Bang Model, protons and electrons are thought to have been created before
atoms, so an enormous amount of energy must have been released during
the formation of the atoms in the Universe. It seems possible that if
the Big Bang Model is correct, then this energy would still be available
to re-ionize large numbers of atoms. Alternatively, it seems possible
that not all protons and electrons combined into atoms after the Big
Bang.
[ a serious flaw with the Big Bang theory which is incomplete anyway and is superseded by my developing Cloud Cosmology which assumes only that we exist or more rigorously that I exist - arclein ]
[ a serious flaw with the Big Bang theory which is incomplete anyway and is superseded by my developing Cloud Cosmology which assumes only that we exist or more rigorously that I exist - arclein ]
Note that the Electric Model does not rely on the Big Bang Model. The Electric Model simply says that we detect ions and electrons everywhere we have looked; so they do
exist, probably in large numbers. Telescopes which “see” in high energy
photons, such as Chandra (X-ray) and EIT, Extreme Ultraviolet Imaging
Telescope on the SOHO solar observation spacecraft, attest to the
presence of ionizing energy sources in the Universe, near and far. To
suggest that mobile ions and electrons can’t exist in large numbers
because, theoretically, there isn’t enough energy to have created them
is as erroneous as arguing that the Universe can’t exist for the same
reason.
3.4 Plasma Research
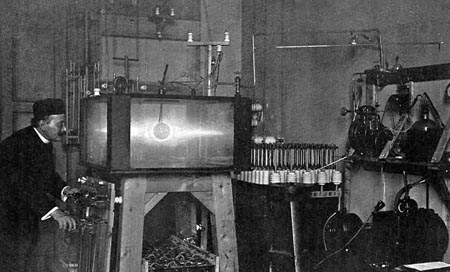
Norwegian scientist Kristian Birkeland (1867-1917) with his Terella (“Little Earth), an evacuated electromagnetic plasma simulator, circa 1904
Although plasma may not be common in
Earth’s biosphere, it is seen in lightning in its many forms, the
northern and southern auroras, sparks of static electricity, spark plug
igniters, flames of all sorts (see Chapter 2, ¶2.6), in vacuum tubes
(valves), in electric arc welding, electric arc furnaces, electric
discharge machining, plasma torches for toxic waste disposal, and neon
and other fluorescent lighting tubes and bulbs.
Plasma behavior has been studied
extensively in laboratory experiments for over 100 years. There is a
large body of published research on plasma behavior by various
laboratories and professional organizations, including the Institute of
Electrical and Electronics Engineers (IEEE), which is the largest
technical professional organization in the world today. The IEEE
publishes a journal, Transactions on Plasma Science.
We will be relying on much of this
research when explaining plasma behavior in the rest of this Guide. One
point to bear in mind is that plasma behavior has been shown to be
scalable over many orders of magnitude. That is, we can test small-scale
examples of plasma in the laboratory and know that the observable
results can be scaled up to the dimensions necessary to explain plasma
behavior in space.
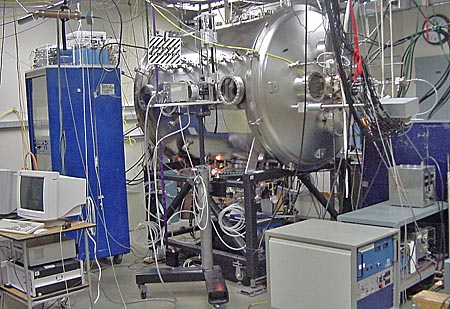
Experimental plasma vacuum chamber in Dr. Paul Bellan’s Plasma Physics Group lab at the California Institute of Technology, USA; circa 2008. Image credit: Cal Tech
3.5 Plasma and Gases
Due to the presence of its charged
particles, that is, ions, electrons, and charged dust particles, cosmic
plasma behaves in a fundamentally different way from a neutral gas in
the presence of electromagnetic fields.
Electromagnetic forces will cause
charged particles to move differently from neutral atoms. Complex
behavior of the plasma can result from collective movements of this
kind.
A significant behavioral characteristic
is plasma’s ability to form large-scale cells and filaments. In fact,
that is why plasma is so named, due to its almost life-like behavior and
similarities to cell-containing blood plasma.
The cellularization of plasma makes it
difficult to model accurately. The use of the term ‘ionized gas’ is
misleading because it suggests that plasma behavior can be modeled in
terms of gas behavior, or fluid dynamics. It cannot except in certain
simple conditions.
Alfvén and Arrhenius in 1973 wrote in Evolution of the Solar System:
“The basic
difference [of approaches to modeling] is to some extent illustrated by
the terms ionized gas and plasma which, although in reality synonymous,
convey different general notions. The first term gives an impression of a
medium that is basically similar to a gas, especially the atmospheric
gas we are most familiar with. In contrast to this, a plasma,
particularly a fully ionized magnetized plasma, is a medium with
basically different properties.”
3.6 Conduction of electricity
Plasma contains dissociated charged particles which can move freely. Remembering that, by definition, moving charges constitute a current,
we can see that plasma can conduct electricity. In fact, as plasma
contains both free ions and free electrons, electricity can be conducted
by either or both types of charge.
By comparison, conduction in a metal is
entirely due to the movement of free electrons because the ions are
bound into the crystal lattice. This means plasma is an even more
efficient conductor than metals, as both the electrons and their
corresponding ions are considered free to move under applied forces.
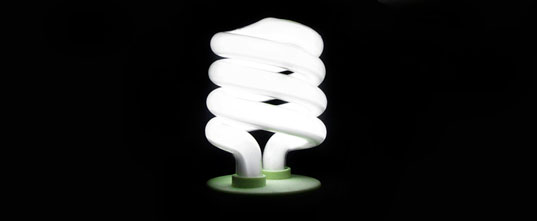
The efficiency of plasma conduction in compact fluorescent lights has rapidly replaced most metal filament (resistance heating) light sources
3.7 Electrical Resistance of Plasmas
In the Gravity Model, plasma is often assumed for simplicity to be a perfect conductor with zero resistance. However, all plasmas have a small but nonzero resistance.
This is fundamental to a complete understanding of electricity in
space. Because plasma has a small nonzero resistance, it is able to
support weak electric fields without short-circuiting.
The electrical conductivity of a
material is determined by two factors: the density of the population of
available charge carriers (the ions and electrons) in the material and
the mobility (freedom of movement) of these carriers.
In space plasma, the mobility of the
charge carriers is extremely high because, due to the very low overall
particle density and generally low ion temperatures, they experience
very few collisions with other particles. On the other hand, the density
of available charge carriers is also very low, which limits the
capacity of the plasma to carry the current.
[ I expect that this is not entirely true but may need to be observed in the ultraviolet. - arclein ]
Electrical resistance in plasma, which depends on the inverse of the product of the charge mobility and the charge density, therefore has a small but nonzero value.
Electrical resistance in plasma, which depends on the inverse of the product of the charge mobility and the charge density, therefore has a small but nonzero value.
Because a magnetic field forces charged
particles moving across the field to change direction, the resistance
across a magnetic field is effectively much higher than the resistance
in the direction of the magnetic field. This becomes important when
looking at the behavior of electric currents in plasma.
Although plasma is a very good conductor, it is not a perfect conductor, or superconductor.
3.8 Creation of Charge Differences
Over a large enough volume, plasma tends
to have the same number of positive and negative charges because any
charge imbalance is readily neutralized by the movement of the
high-energy electrons. So the question arises, how can differently
charged regions exist, if plasma is such a good conductor and tends to
neutralize itself quickly?
On a small scale, of the order of tens
of meters in a space plasma, natural variations will occur as a result
of random variations in electron movements, and these will produce small
adjacent regions where neutrality is temporarily violated.
On a larger scale, positive and negative
charges moving in a magnetic field will automatically be separated to
some degree by the field because the field forces positive and negative
charges in opposite directions. This causes differently charged regions
to appear and to be maintained as long as the particles continue to move
in the magnetic field.
Separated charge results in an electric
field, and this causes more acceleration of ions and electrons, again in
opposite directions. In other words, as soon as some small
inhomogeneities are created, this rapidly leads to the start of more
complex plasma behavior.
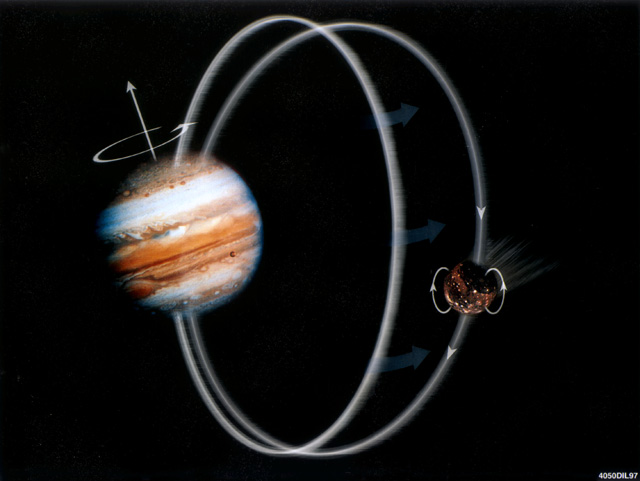
Moving through Jupiter’s intense magnetic field creates strong charge separation (voltage differential) and a resulting electrical current in a circuit of some 2 trillion watts power flowing between Io and Jupiter’s polar areas
Over all scales, the signature
filamentation and cellularization behavior of plasma creates thin layers
where the charges are separated. Although the layers themselves are
thin, they can extend over vast areas in space.
3.9 Important Things to Remember About Plasma Behavior
The essential point to bear in mind when
considering space plasma is that it often behaves entirely unlike a
gas. The charged particles which are the defining feature of a plasma
are affected by electromagnetic fields, which the particles themselves
can generate and modify.
In particular, plasma forms cells and
filaments within itself, which is why it came to be called plasma, and
these change the behavior of the plasma, like a feedback loop.
Plasma behavior is a little like fractal
behavior. Both are complex systems arising from comparatively simple
rules of behavior. Unlike fractals, though, plasma is also affected by
instabilities, which add further layers of complexity.
Any theoretical or mathematical model of
the Universe that does not take into account that complexity, is going
to miss important aspects of the system’s behavior and fail to model it
accurately.
end of Chapter 3
No comments:
Post a Comment