The most important form of radiation in the universe is a single line in the ultraviolet. It is the natural wavelength associated with Dark Matter.
The rest of the radiation in the universe is produced by decay and that includes natural light. After all that is produced by an electron decaying to a lower orbit
There are then three dominant tiers of radiation production. we understnad the third tier which is all physical matter as we know it. The second tier is produced by the decay process that produces electrons and its associated complex of related particles which may be simple but that looks to be a forlorn hope. The third tier is that of neutral neutrino creation.
.
Essential Guide to the EU – Chapter 11 Radiation
11.1 Light
https://www.thunderbolts.info/wp/2012/03/30/essential-guide-to-the-eu-chapter-11/
Sunrise illuminates the landscape with visible (and invisible) light
Visible light ranges from red
through yellow and green to blue and violet. Newton was the first to
discover that white light is a mixture of all the colors. White light
can be split into its component colors by diffraction through a prism, which ‘bends’ each color by a different amount. A diffraction grating
is often used in astronomy because tiny or dim light sources suffer
less energy loss in reflecting from a hard, ruled surface than is lost
traveling through a glass prism.
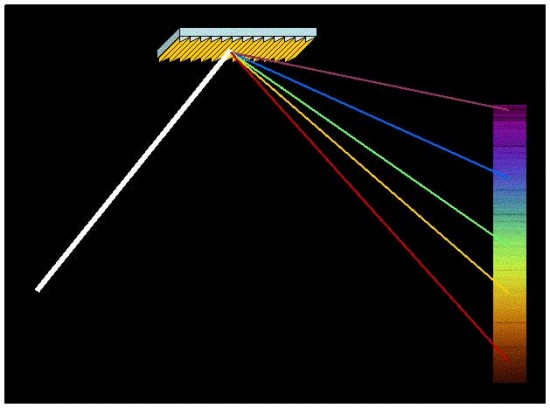
White light can be separated into its component colors by reflecting from a finely-grooved flat metal grating, courtesy NASA, Jet Propulsion Laboratory
Eventually, Maxwell, who defined the
Electromagnetic Field equations, proved that light was in fact made up
of electromagnetic (EM) waves. Each color of visible light has a
characteristic frequency and wavelength. As with all waves, the product
of frequency and wavelength give the velocity of the wave. Obviously,
light travels at the velocity of light, but Maxwell was able use his
equations to shown that all electromagnetic waves travel with the velocity of light, and so light must also be an electromagnetic wave.
Visible light represents only a small
part of all the possible frequencies or wavelengths. The whole range is
known as the electromagnetic spectrum.
11.2 The Spectrum
Although the spectrum is continuous,
each region of the spectrum has been named after a typical type of wave
for that part of the spectrum.
Starting with the lowest-frequency,
longest-wavelength waves, the spectrum runs from radio waves through
microwaves (as in ovens), terahertz
radiation (a recent development in military communications), infrared
(as in heaters), the visible spectrum (Red, Orange, Yellow, Green, Blue,
Indigo, Violet), ultraviolet (tanning and forensic lamps, materials
analysis), X-rays (internal medical images), and up to Gamma rays
(cancer treatments).
The spectrum is shown in the following
diagram. Note that the visible spectrum is only a small part of the
whole spectrum. When the Gravity Model was formulated, scientists could
only see visible light from the heavens.
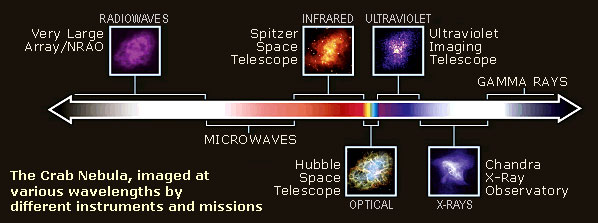
Diagram of the electromagnetic spectrum, with images of the Crab nebula showing how it would “look” if we could see beyond the limits of our eyes’ light sensitivity range, courtesy NASA
In the 20th century, and especially
since the start of the Space Age in the 1950s, instruments have been
developed which allow scientists to detect virtually all wavelengths.
The amount of information available has increased exponentially.
Observations are often surprising because what is seen in the visible is
seldom anything like what is found at other wavelengths.
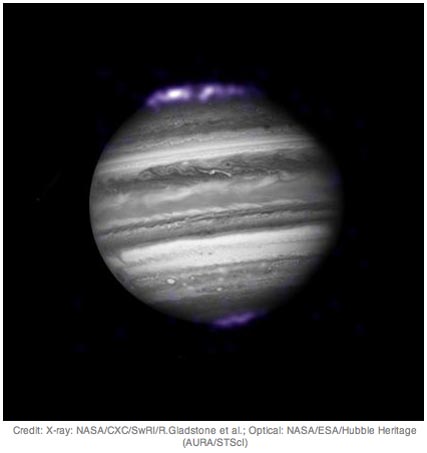
Jupiter as seen in optical wavelengths (grayscale), with its auroras radiating in invisible X-ray radiation (mapped to visual violet as “false color” to appear visible to us). Image credit: NASA / Chandra X-ray Telescope
11.3 Radiation
Radiation is the process whereby energy
is emitted by one body, transmitted through a medium or through space,
and eventually absorbed by another body. The emitting and absorbing
bodies can be as small as individual atoms or even subatomic particles
like electrons.
Electromagnetic waves are the means whereby the energy is transmitted. In other words, all radiation is electromagnetic.
This means that the mode of transmission
of radiation involves oscillating electric and magnetic fields which
carry the energy similar to the way that vibrations on a string carry
energy along the string. Since the speed of transmission of vibrational
energy is constant for a given medium, and that value is equal to the
frequency of the vibrating wave times the wavelength (frequency times
wavelength = velocity), if you know the frequency one can solve for the
wavelength at that frequency, and vice versa.
The spectrum represents the range of
possible frequencies or wavelengths of the radiation. As the frequency
increases, the amount of energy carried by the wave also increases in
proportion to the frequency. Ionizing radiation is radiation which
carries sufficient energy to ionize atoms. In general terms, frequencies
from radio to the visible do not carry enough energy for this, while
ultraviolet, X-ray and Gamma ray radiation can ionize. As noted
previously, ionization energy varies with different elements and
molecules.
Radiation is emitted whenever a charged
particle undergoes acceleration. Remembering that a change of direction
is also an acceleration because the direction of the velocity is
changing, then every charged particle that experiences a change of
direction will emit radiation.
Current theories explain this emission
in terms of the emission of a photon, or packet of energy. A photon has
no mass but carries the radiated energy in the form of electromagnetic
waves. A photon behaves both like a wave and like a particle. Which mode
is more significant will depend on the circumstances.
To summarize:
- Radiation is emitted by all charged particles undergoing acceleration.
- All radiation involves electromagnetic waves.
- Radiation transmits energy.
- The spectrum represents the range of possible frequencies or wavelengths of the radiation.
11.4 Thermal radiation
Thermal radiation is radiation emitted
from a surface of a body or region of particles due to the temperature
of the body or region.
Temperature is a measure of the thermal
energy contained in a body. The thermal energy causes the charged
particles inside the atoms of the body to vibrate in a random fashion.
They will therefore emit radiation over a range of frequencies.
Similarly, a region of plasma can have a temperature.
A proportion of this radiation is
emitted from the surface of the body or region as heat (infrared
radiation). Actually, all matter with any internal thermal
motion radiates EM energy: the colder it is, the longer the wavelength
it radiates. Cold interstellar dust will radiate terahertz, or
sub-millimeter wavelength radiation, starting at a temperature of only
about 10 Kelvin.
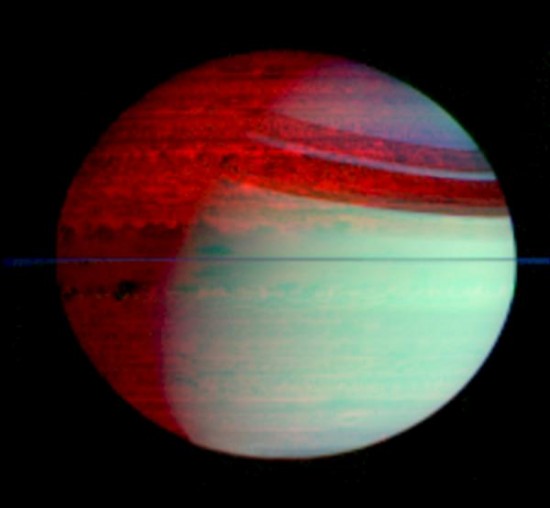
Cold Saturn’s deep nighttime hemisphere imaged in infrared radiation by Cassini’s thermal/optical mapping spectrometer in 2006. Image courtesy, NASA / JPL / Cassini Imaging Team
Because of the random nature of the vibrations over a large number of particles, the emitted radiation will have a range of frequencies, or wavelengths. Statistical analysis shows that in an ideal situation the energy emitted at any one wavelength is a function of that wavelength. This is known as Planck’s Law and is shown graphically below for a range of temperatures. The radiation emitted in this ideal situation is known as Blackbody radiation, which simply means that it has the distribution pattern that would be expected from a perfect emitter in thermal equilibrium. (Diagram source: Wikipedia ‘blackbody‘ article)
Ideal blackbody radiation for 3 temperatures, showing that the peak wavelength emitted shifts to higher frequencies (shorter wavelengths) with increasing temperature. Image credit: Wiki Commons
The graphs show that for any one
temperature there is one wavelength at which the greatest amount of
energy is emitted. As the temperature increases, the wavelength of the peak energy decreases. This is defined by another law known as Wien’s Law. Note that the red line has a lower temperature and lower area under its curve than the hotter blue line.
The area under any one temperature curve
gives the total amount of energy emitted at that temperature, per unit
area. The total energy emitted per unit area depends only on the
temperature. This is known as the Stefan-Boltzmann Law.
If the pattern of emitted radiation from
any source is distributed in the form given by Planck’s Law, then the
emission is assumed to be due to random thermal movements of particles
in the source. We then say that the radiation is thermal radiation. All
this means is that the radiation has a distribution of wavelengths or
frequencies which come from the random thermal vibrations of particles.
The radiation itself is electromagnetic radiation like any other
radiation.
If we find that radiation is thermal,
then we can determine the temperature of the source by comparing the
emitted radiation curve to the ideal ‘Black Body’ curves. This means we
can determine the temperature of distant objects if the radiation they
emit is thermal radiation. Stars have been found to have a spectrum
which approximates a blackbody distribution, so the color temperature of stars can be inferred from their spectra.
Non-thermal radiation is simply
radiation which is not emitted in a thermal pattern. It must therefore
be generated by other methods than random temperature-induced motions of
the particles in a system in thermal equilibrium.
That is not to say that temperature
cannot play a part in causing these other patterns of radiation; it is
simply that the system or body that is emitting the radiation is not in
thermal equilibrium. In other words, energy is being exchanged with the
system so that its temperature is changing with time. This will alter
the ideal Black Body pattern of radiation and mean that it is not
possible to assign a temperature to the body.
Alternatively, the radiation may be
emitted by individual particles undergoing acceleration caused by means
other than random collisions with other particles.
11.5 Optical Radiation in the Cosmos
Radiation in the cosmos is common in the
visible and radio wavelengths. In the optical region, the majority of
radiation is generated by electrons jumping to new orbits within an atom
(bound-bound transitions), free electrons recombining with ions to form
neutral atoms (free-bound transitions) and electrons being decelerated
by interaction with other material (free-free radiation).
The bound-bound transitions are a source
of both emission lines and absorption lines in the spectrum. Each
chemical element has a range of energies associated with the range of
possible electron orbits around the nucleus for that element. As an
electron jumps from one orbit to another, energy in the form of
radiation is either absorbed or given off. The energy represents the
difference in the orbital energies and so is precisely defined for each
possible jump between levels.
Because the energy of a photon is
proportional to its frequency, these energy differences will result in
radiation with a defined set of frequencies for each element. If the
radiation energy emitted from an element is plotted for each frequency
in the spectrum, then there will be sharp peaks in the graph at these
frequencies. These are known as emission lines in the spectrum.
On the other hand, if light with a broad
range of frequencies passes through a medium containing certain
elements or molecules, those elements are found to absorb energy at
their characteristic frequencies. The resulting spectrum will be missing
those frequencies, and dark lines will appear. These are known as absorption lines.
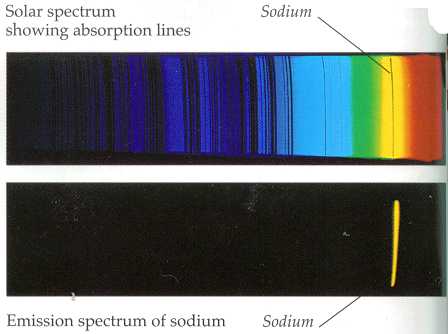
Lower, the primary yellow-light sodium emission line, from heated sodium atoms. Above is an absorption spectrum where light from a blackbody-like source (such as a star) has passed through a volume of space containing sodium on its way to the observer or imaging instrument. The black line is where the sodium atoms have absorbed light preferentially at that frequency, leaving a dark line at that “color”. Image courtesy of ThinkQuest (www.thinkquest.org), funded by the Oracle Education Foundation
Here is an introduction to blackbody radiation and absorption lines and continuum radiation. And here is more to explore in the broader context of spectroscopy.
As an example, if an element is heated
in a star’s interior, then it will give off its characteristic radiation
which we can detect as bright emission lines on Earth. On the other
hand, if broadband light has passed through an absorbing medium between
the observer and the light source, then we can determine the elements in
that medium by looking for the dark absorption lines.
Free-bound transitions occur when
electrons are captured by ions and result in release of energy as
recombination occurs. The amount of energy released is dependent on the
element formed and the orbit that the electron occupies. As in the
bound-bound transition, certain frequencies may dominate.
Free-free radiation occurs when
electrons undergo a non-capture collision with an ion or a charged dust
particle in the plasma. The electron’s trajectory will be changed as it
passes near the other particle, and so it will give off radiation, some
of which may be in the visible spectrum.
11.6 Radio Radiation in the Cosmos
Radio wavelengths are important because
many radio waves can penetrate the Earth’s ionosphere and so can be
detected by ground-based radio telescopes.
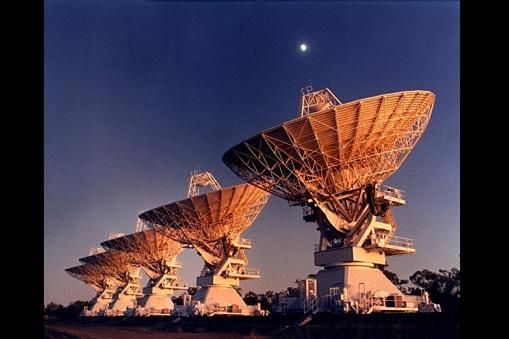
Radio telescope array in New South Wales, Australia. Image credit, University of Waikato and Commonwealth Scientific and Industrial Research Organisation (CSIRO)
Some radio radiation in the cosmos is a
result of the collective behavior of large numbers of electrons in a
plasma. If the plasma is sufficiently dense, then the electrons can
oscillate collectively with a frequency known as the plasma frequency,
which depends only on the density of the electrons in the region. These
oscillations generate radiation in the usual way.
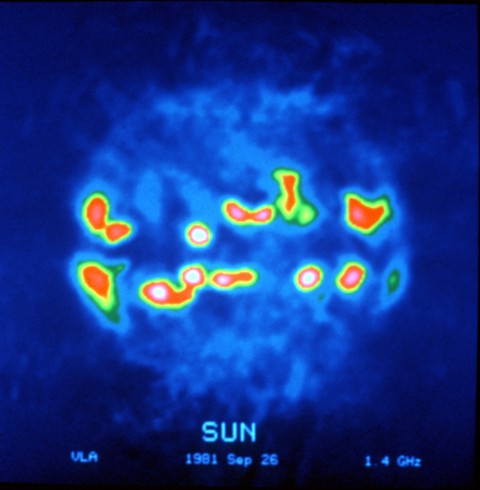
The Sun, as seen (in false color) at a radio wave frequency of 1.4 GHz, with strong emissions at the active regions in the solar equatorial belt. Image courtesy of the National Radio Astronomy Observatory (NRAO/AUI)
This type of radiation often occurs when
a beam of electrons, for example, as might be generated by acceleration
through a double layer, passes through a region of neutralizing plasma.
There are other radio frequency
radiation generating mechanisms where a magnetic field is present. These
include cyclotron radiation (where electrons have non-relativistic
velocities), Magneto-Bremsstrahlung radiation (where electrons have
mildly relativistic velocities), and synchrotron radiation (where
electrons have relativistic velocities).
Synchrotron radiation is produced by
electrons spiraling along the direction of a magnetic field, such as
occurs in Birkeland Currents (image in 11.3 above). The centripetal
acceleration causes the radiation. Again, the radiation can occur at all
frequencies across the spectrum.
In astrophysics, non-thermal radio
emission is, in most cases, synchrotron radiation. This is true for
galactic radio emissions, supernovae envelopes, double radio galaxies,
and quasars. Additionally, the Sun and Jupiter both produce sporadic
synchrotron emissions.
Synchrotron emission can also generate optical frequencies, such as are seen in the Crab Nebula and the M87 ‘jet’. The Crab Nebula (short YouTube video) also emits quantities of X ray synchrotron radiation.
The analysis of a synchrotron spectrum
can give information on the source of the relativistic electrons, which
may have a bearing on the origin of cosmic rays, X rays, and Gamma rays
in space. Synchrotron radiation is also evidence for the existence of
extensive magnetic fields in space and for the conversion, storing, and
releasing of large amounts of energy in cosmic plasmas, including
galactic jets. More details on synchrotron radiation here for the interested explorer.
Z-pinches can also generate synchrotron radiation as a result of the v × B force.
Radio astronomy can therefore extend the
range of information available to us well beyond that derivable from
visual telescopes alone. Detection of higher energy spectra such as
X-rays can take this knowledge a stage further.
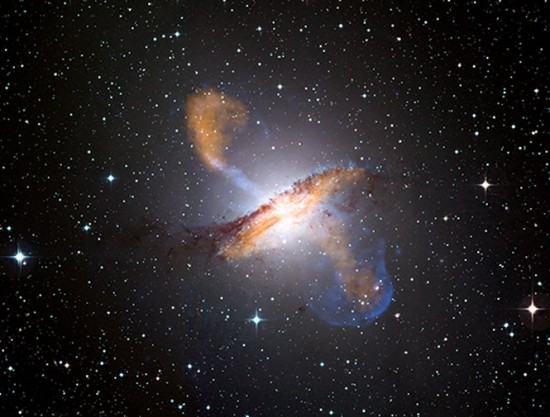
Active galaxy Centaurus A (NGC 5128) , composite image in sub-millimeter (radio plumes; orange), visible (white) and X-ray radiation (blue). Galaxy’s diameter is approximately 200,000 light years; estimated distance 10 million light years. Credit: X-ray: NASA/CXC/Cfa/R. Kraft et al.: sub-millimeter: MPIfR/ESO/APEX/A.Weiss et al. Optical: ESO/WFI
A short NASA video on this galaxy is here, although the EU does not speculate about black holes’ causing galactic jets and their resultant radiation.
In all cases we find that plasmas and
electric currents within them are excellent emitters of radiation
because, as we have seen, electricity in plasma is extremely good at
accelerating charged particles, via the electric fields across double
layers, which particles then emit the radiation. Interesting research
arXiv article posted here.
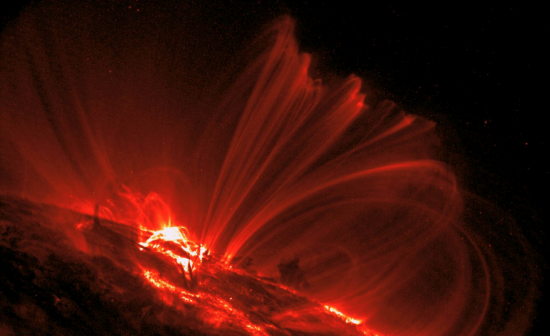
Arcades of solar plasma filaments in the lower corona of the Sun are revealed in far ultraviolet light by TRACE instruments, courtesy The TRACE Picturebook, NASA
This efficient production of radiation
by electrical mechanisms seems to be a much more likely source of most
of the radiation detected in space than are the huge amounts of Dark
Matter and super-dense matter necessary to explain particle acceleration
using only gravity.
Of course, ‘magnetic reconnection’, the
alleged breaking and reconnection of magnetic field lines, is also often
invoked to explain this type of evidence in the Gravity Model. As we
have seen, this is simply impossible because magnetic field lines don’t
have a physical existence any more than lines of longitude do.
End of Chapter 11
No comments:
Post a Comment