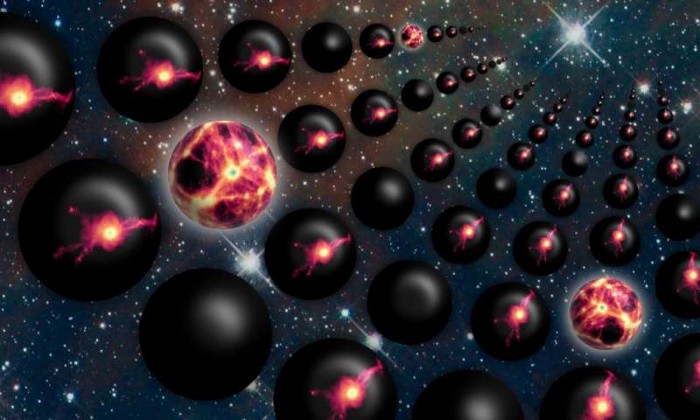
first off, we can imagine and define mathematical infinity and it simply does not exist in our empirical universe. The good news for mathematician's is that it only matters with cyclic functions and until I came along, we only had sine and cosine to worry about.
The fact that an end point exists gives us gravity. just saying.
What bothers me is that all the Galaxies more or less look to be the same size and scale providing us a start point. Are we really getting that right? And is consciousness finite at all or can that idea even apply?
Ask Ethan: Is the Universe finite or infinite?
The Universe’s history, from cosmic inflation to the Big Bang to the present, is known. But whether it’s infinite or not is still a mystery.
In inflationary cosmology, the volume that space occupies is stretched to an inconceivably large volume in a tiny fraction of a second, and wherever inflation ends, a hot Big Bang (and a Universe like ours) ensues. This creates a virtual "infinity of worlds," but the number is likely not quite infinite after all.
Credit: Jaime Salcido/EAGLE Collaboration
KEY TAKEAWAYS13.8 billion years ago, our Universe as we know it began with the hot Big Bang, which gave rise to a primordial soup of particles and antiparticles that led to the planets, stars, and galaxies we know today.
The hot Big Bang itself was set up by a preceding phase known as cosmic inflation, but only the final tiny fraction-of-a-second gets imprinted onto our observable Universe.
What we can observe about the Universe is finite, but what about the unobservable parts that lie beyond it: are they finite or infinite? What the data can tell us is limited, but here’s what we think and why.
One of the most important imprints that persists from the earliest times is the initial spectrum of density fluctuations: the “imperfections” that the Universe was born with, at the start of the hot Big Bang, that seeded any and all departures from perfect uniformity. On the largest of cosmic scales, the Universe is both isotropic and homogeneous, where:isotropic tells us that the Universe appears the same in all directions, regardless of what orientation you’re facing,
and homogeneous tells us that the Universe appears the same in all locations, regardless of where you place yourself.
If you were to take a ladle that had a size of around 10 billion light-years (or larger) and “dipped it into” any region of our Universe today, you’d find that it pulled out the same amount of “stuff,” including all forms of matter and energy, regardless of where you dipped it. Well, almost the same amount: the “least dense” regions would be about 1-part-in-30,000 less dense than average, while the “most dense” regions would be about 1-part-in-30,000 more dense than average.

In a Universe that comes to be dominated by dark energy, there are four regions: one where everything within it is reachable and observable, one where everything is observable but unreachable, one where things will someday be observable but aren’t today, and one where things will never be observable. The labeled numbers correspond to our consensus cosmology as of 2024, with boundaries of 18 billion light-years, 46 billion light-years, and 61 billion light-years separating the four regions. On scales of ~10 billion light-years and larger, the Universe is almost perfectly uniform.Credit: Andrew Z. Colvin/Wikimedia Commons; annotations: E. Siegel
On smaller cosmic scales — the scales of galactic filaments, clusters and voids, individual galaxies and the intergalactic medium, etc. — however, the variations that we see in density are much, much grander. Here on planet Earth, for example, the density of our world is approximately ~1030 times the average cosmic density: about as non-uniform as you can get!
There’s a physical reason behind why the Universe is so uniform on large scales, but so non-uniform on smaller scales: the combination of gravitation along with cosmic expansion.
Gravity, as a force, has an important limit: the speed at which it propagates is limited by c, the speed of light in a vacuum. (Yes, the speed of gravity and the speed of light are identical.) Objects can only experience the gravitational effects of matter and energy that are close enough so that a sufficient amount of time has passed for the force to propagate from one source to the other; objects more distant than that cannot yet experience gravity.
Meanwhile, cosmic expansion causes the distance between objects that are not (yet) gravitationally bound together to increase, so that objects separated by small distances can “feel” the gravitational force sooner in cosmic history than objects separated by great distances.
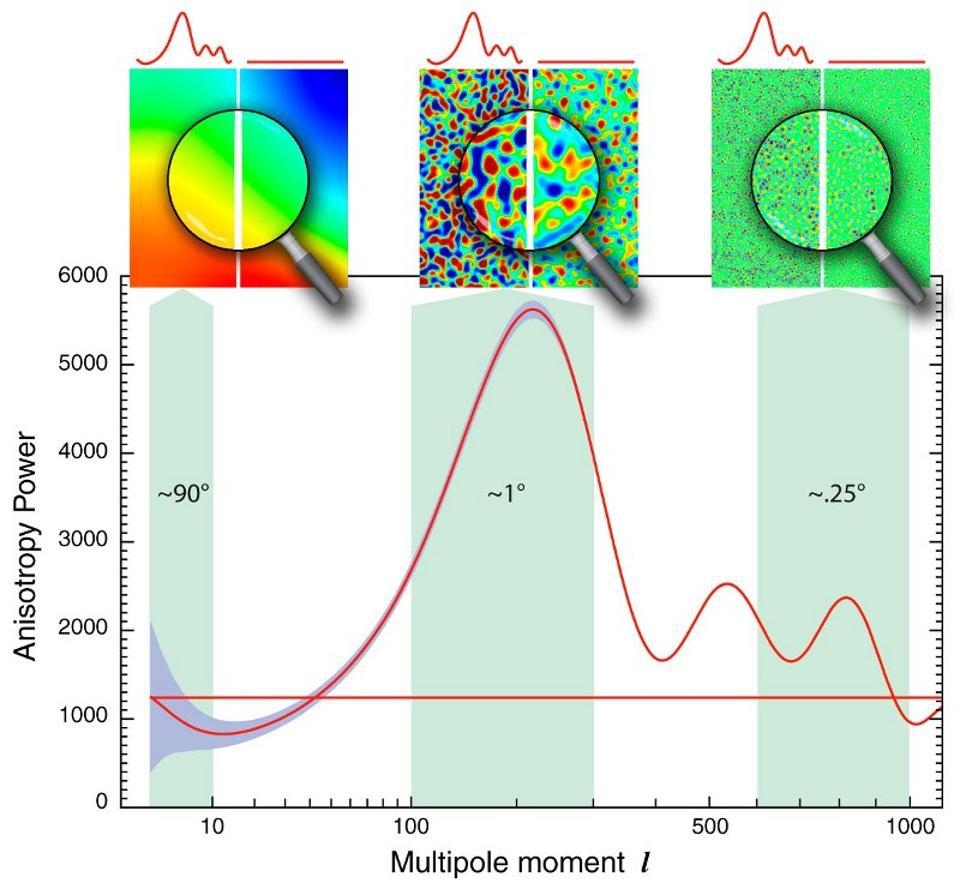
The fluctuations in the cosmic microwave background, as measured by COBE (on large scales), WMAP (on intermediate scales), and Planck (on small scales), are all consistent with not only arising from a (slightly tilted, but almost-perfectly) scale-invariant set of quantum fluctuations, but of being so low in magnitude that they could not possibly have arisen from an arbitrarily hot, dense state. The horizontal line represents the initial spectrum of fluctuations (from inflation), while the wiggly one represents how gravity and radiation/matter interactions have shaped the expanding Universe in the early stages.Credit: NASA/WMAP science team
The idea of the hot Big Bang is that the Universe was born expanding very rapidly and was structured in an almost-perfectly uniform fashion on all scales: small, intermediate, and large ones alike. Whatever imperfections existed at the start of the hot Big Bang — overdense regions and underdense regions alike — will gravitationally evolve, with their evolution dependent on all the relevant interactions that take place within those regions. For example:the initially overdense regions will preferentially gravitationally attract the matter around them, drawing it in, while the initially underdense regions tend to give up their matter to their dense surroundings, any radiation in those overdense regions streams out of it, suppressing the growth of structure, while radiation “pushes” normal, charged matter (like protons and electrons) outward, but not dark matter, while larger-scale regions have to wait for longer amounts of time to pass for that first “gravitational infall” to occur.
This means that if we begin with an almost perfectly uniform Universe, but one that was born imprinted with small-magnitude overdensities and underdensities within it, we can expect to see structure forming in a specific way that depends on the types of initial seeds that were planted. In particular, the imprints of these initial seeds show up, today, in two different ways: in the peaks-and-valleys of the temperature fluctuations imprinted early on into the cosmic microwave background (CMB), and in the large-scale clustering of galaxies seen all throughout cosmic history.
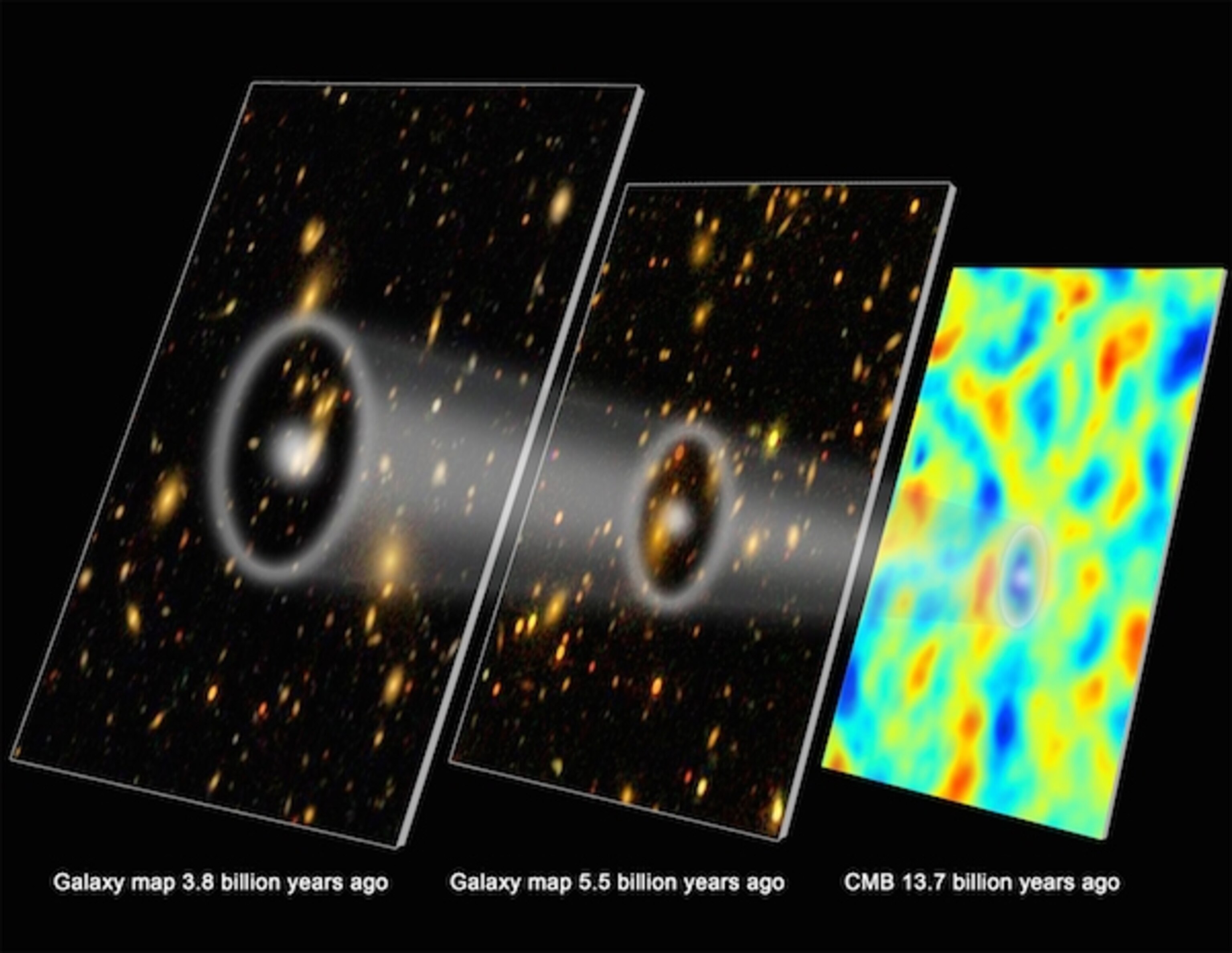
We can look arbitrarily far back in the Universe if our telescopes allow, and the clustering of galaxies should reveal a specific distance scale – the acoustic scale – that should evolve with time in a particular fashion, just as the acoustic “peaks and valleys” in the cosmic microwave background reveal this scale as well. The evolution of this scale, over time, is an early relic that reveals a low expansion rate of ~67 km/s/Mpc, and is consistent from CMB features to BAO features.Credit: E M Huff, the SDSS-III team and the South Pole Telescope team; graphic by Zosia Rostomian
These two observables — the CMB and the large-scale clustering of galaxies — encode tremendous information about our Universe’s history. What they teach us, as of 2024, is remarkable. Here are some of the most important lessons that we can learn from investigating them.The observable Universe, out to the limits of how far away we can observe it (46.1 billion light-years, in all directions), shows no signs of repeating structures or patterns anywhere: evidence that if the Universe is finite and closed, it must be larger than the portion we can see.
The observable Universe possesses a spatial curvature that is almost completely perfectly flat, indicating that if it is, say, a higher-dimensional analogue of a sphere (a hypersphere), its minimum size is at least ~400 times larger than the portion of it that we can see.
And that, in order to get the observed structures and features we see, both in the CMB and in the way galaxies and other forms of matter clump and cluster together (i.e., the matter power spectrum), the Universe must have been born with a specific spectrum of initial “seed” density imperfections: one that began as almost perfectly scale invariant (i.e., with the same magnitude of Gaussian, random fluctuations on all scales), but where the “large scales” have about a ~3% larger-magnitude set of fluctuations than “small scales” from a cosmic perspective.
By looking at this evidence, we can draw a number of important conclusions about both the size of the Universe and the earliest moments — even a tiny fraction-of-a-second prior to the hot Big Bang — that we can say something physically meaningful about.
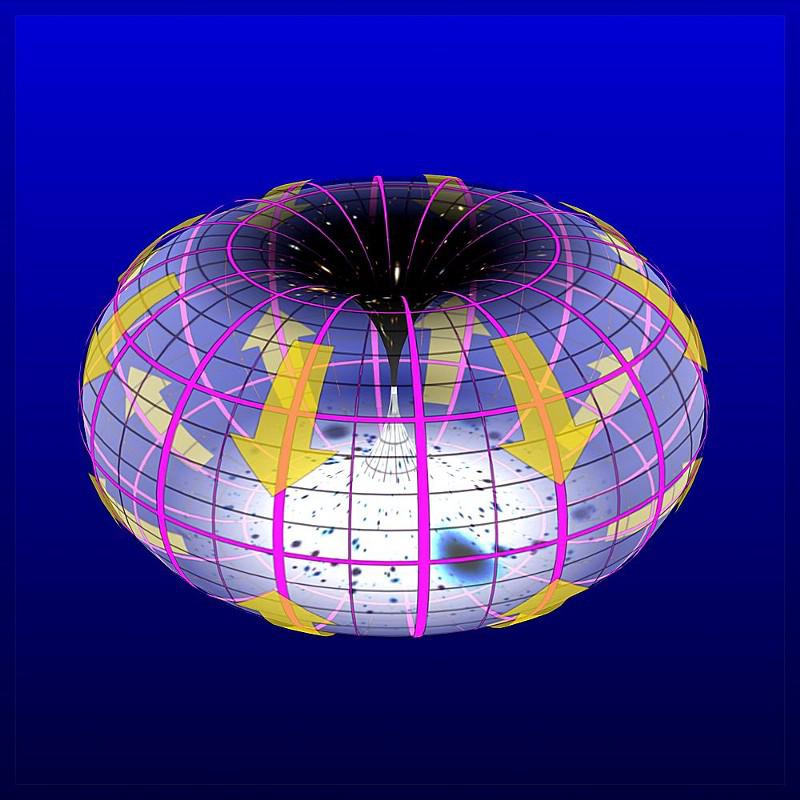
A visualization of a 3-torus model of space, where our observable Universe could be just a small portion of the overall structure. Similar to imagining our Universe (or any three-dimensional space) being enclosed by a two-dimensional boundary, our three-dimensional space may in fact be the boundary around a higher-dimensional space. Although there are constraints on the properties and number of such extra dimensions, the possibility cannot be ruled out. However, the lack of repeating structures and the spatial flatness of the Universe tells us important information about how much larger than the visible part of the Universe, at least, the unobservable Universe must be.Credit: Bryan Brandenburg/Wikimedia Commons
For the Universe as a whole, the observed spatial flatness tells us that it cannot be severely positively curved (like a sphere) nor can it be severely negatively curved (like a horse’s saddle or a Pringles potato chip). Instead, it’s spatially flat, as evidenced by the sizes and scale of fluctuations seen imprinted in the CMB and the evolution of the acoustic scale (the peaks and valleys observed in galaxy clustering) across cosmic time. The observed lack of repeating structures places very strong constraints on a Universe with a non-trivial topology, like a donut-shaped Universe, where we would expect that you could look in one direction and see an object, and in the opposite direction also see that same object. We can’t say whether the Universe is finite or infinite based on this alone, but we can say:if it is finite, the size of the Universe is larger than the size of the observable Universe,if the Universe is closed and curved (like a sphere), the size of the unobservable Universe must be at least ~400 times the size of the observable part, and that if the Universe exhibits a non-trivial topology, where it has a finite extent, it is observably indistinguishable from an infinitely large, unbounded Universe.
However, the observed spectrum of fluctuations, combined with the observed spatial flatness and observed large-scale uniformity of the Universe, paints a very interesting picture of our cosmic origin: one that began not with the hot Big Bang, but one that was set up previously by a period of cosmic inflation.

The quantum fluctuations inherent to space, stretched across the Universe during cosmic inflation, gave rise to the density fluctuations imprinted in the cosmic microwave background, which in turn gave rise to the stars, galaxies, and other large-scale structures in the Universe today. This is the best picture we have of how the entire Universe behaves, where inflation precedes and sets up the Big Bang. Unfortunately, we can only access the information contained inside our cosmic horizon, which is all part of the same fraction of one region where inflation ended some 13.8 billion years ago.Credit: E. Siegel; ESA/Planck and the DOE/NASA/NSF Interagency Task Force on CMB research
According to inflation, the Universe wasn’t born in a hot, dense, almost-perfectly uniform, rapidly expanding state, but rather transitioned into that state from a pre-existing one: a sparse, empty state where space was filled not with quanta, but with a form of energy inherent to space itself. Similar to today’s dark energy but billions upon billions upon billions of times stronger, this phase:stretched the Universe to a state that was indistinguishable from flat, removed any pre-existing high-energy relics by inflating them away, and created a uniform set of conditions that were the same everywhere,
with a nearly scale-invariant spectrum of imperfections, or density fluctuations, superimposed atop the uniform background.
Unlike the modern expanding Universe, which has grown to an (observable) size of ~46.1 billion light-years over the span of 13.8 billion years, an inflationary Universe grows much, much larger in tiny periods of time. During inflation, the scale of the Universe doubles — in all three dimensions: length, width, and depth — with every tiny fraction of a second, something like 10-35 seconds, that elapses. After 10-34 seconds have elapsed, the Universe has doubled in size ten times over; after 10-33 seconds, it has doubled 100 times over; after 10-32 seconds, it’s doubled 1000 times over. These numbers are important, because it only takes about ~300 “doublings” to go from something that’s smaller than the Planck scale, or the minimum scale at which physics makes sense, to something that’s larger than the entire observable Universe.
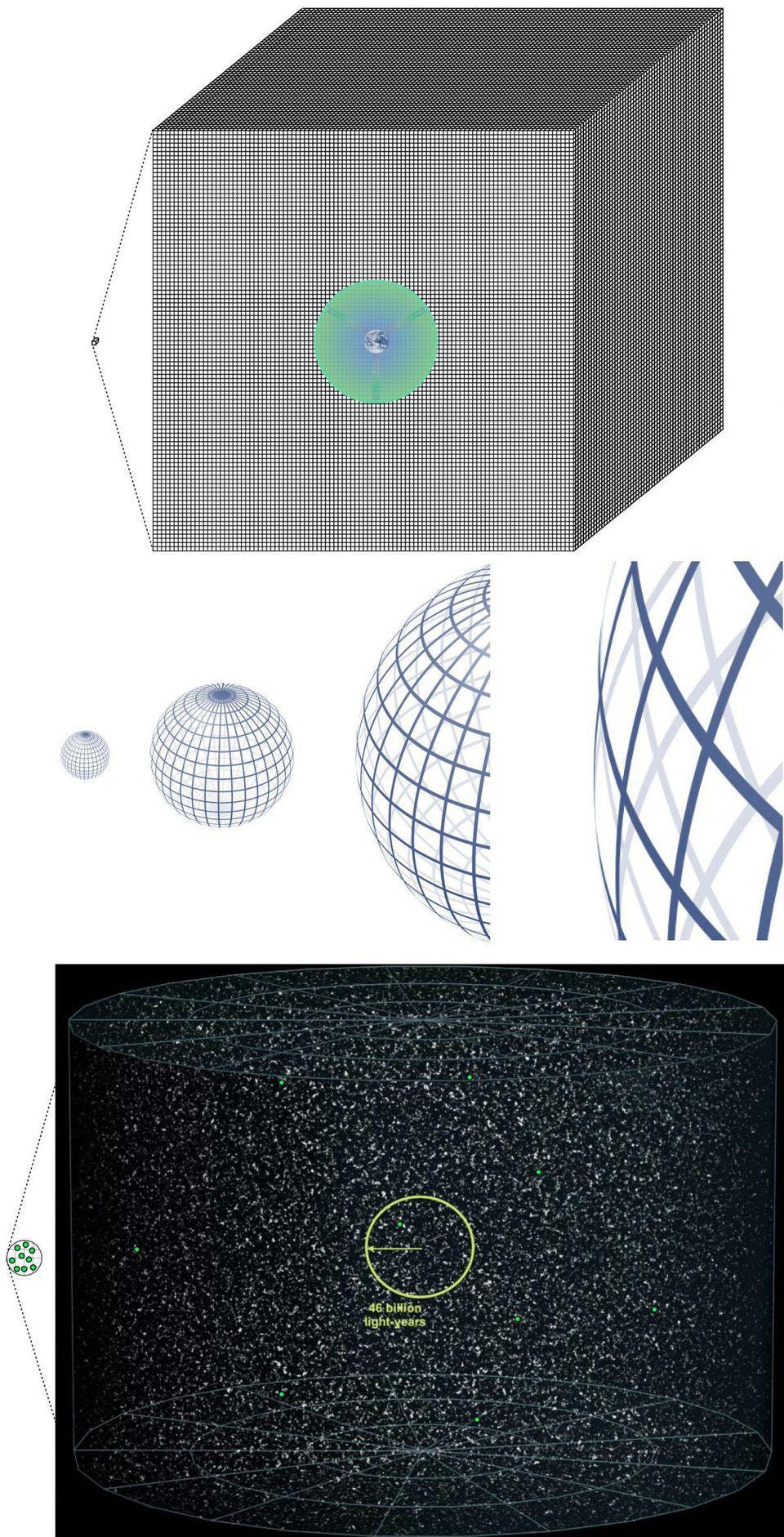
In the top panel, our modern Universe has the same properties (including temperature) everywhere because they originated from a region possessing the same properties. In the middle panel, the space that could have had any arbitrary curvature is inflated to the point where we cannot observe any curvature today, solving the flatness problem. And in the bottom panel, pre-existing high-energy relics are inflated away, providing a solution to the high-energy relic problem. This is how inflation solves the three great puzzles that the Big Bang cannot account for on its own.Credit: E. Siegel/Beyond the Galaxy
What inflation then gives us — maddeningly, to many seeking to understand the Universe — is a story that only describes the very few final “doublings” that occurred, because any information that occurred prior to it automatically gets erased, or stretched/expanded away to unobservably large scales, by the nature of inflation itself.
This point is not often sufficiently appreciated, by laypersons and astrophysicists alike. Inflation gives us a story, and a framework, for understanding how the hot Big Bang was set up, and why it began with the initial conditions that it did. These conditions include:a spatially flat Universe, with the same temperature and uniform density everywhere, with no leftover, ultra-high-energy relics, with an almost scale-invariant, but slightly tilted (with a preference for large-scales) spectrum of initial seed fluctuations, that are adiabatic (and not isocurvature) in nature, including fluctuations that appear on scales larger than the cosmic horizon at any given point in time (a.k.a., superhorizon fluctuations), where inflation led to a hot Big Bang that has a maximum (reheat) temperature that was at least a few orders of magnitude (perhaps a factor of ~1000) lower than Planck-scale temperatures.
All of this comes from the final few hundred “doublings” of the end stages of cosmic inflation, and is independent of what happened prior to those doublings.

During cosmological inflation, the space contained in the inflationary region grows exponentially, doubling in all three dimensions with each tiny fraction-of-a-second that passes. Where inflation ends, a hot Big Bang ensues. But due to quantum effects, each region where a Big Bang occurs will be surrounded by more inflating, exponentially expanding space, ensuring that no two regions where hot Big Bangs occur ever collide, intersect, or overlap.Credit: Kavli IMPU
This leaves us not with a knowledge gap, but rather a knowledge “cliff,” where whatever occurred prior to these final fractions-of-a-second of inflation is fundamentally unknowable, as its history has been erased from the parts of the Universe that are observable, now and forever into the future, by us. Inflation could have lasted for less than 10-32 seconds, or for one second, or for trillions of years, and the Universes that we would observe today would look no different, in any way, between these three scenarios.
There is a reason that many theoretical physicists talk about the Universe as likely being finite rather than infinite, however, and the reason is this: inflationary spacetimes, within the framework of the laws of physics as we understand them (i.e., within the spacetime general relativity), have a property that says they cannot be “past-timelike complete.” In other words, if you ask the question, “Could inflation have gone on for an infinite amount of time prior to our Universe emerging?” the answer that comes back is no.
Something must have preceded the inflationary phase and allowed it to begin. Almost everything is unknown (aside from some consistency conditions) about that state, including whether it had a moment of origin or was eternal to the past.
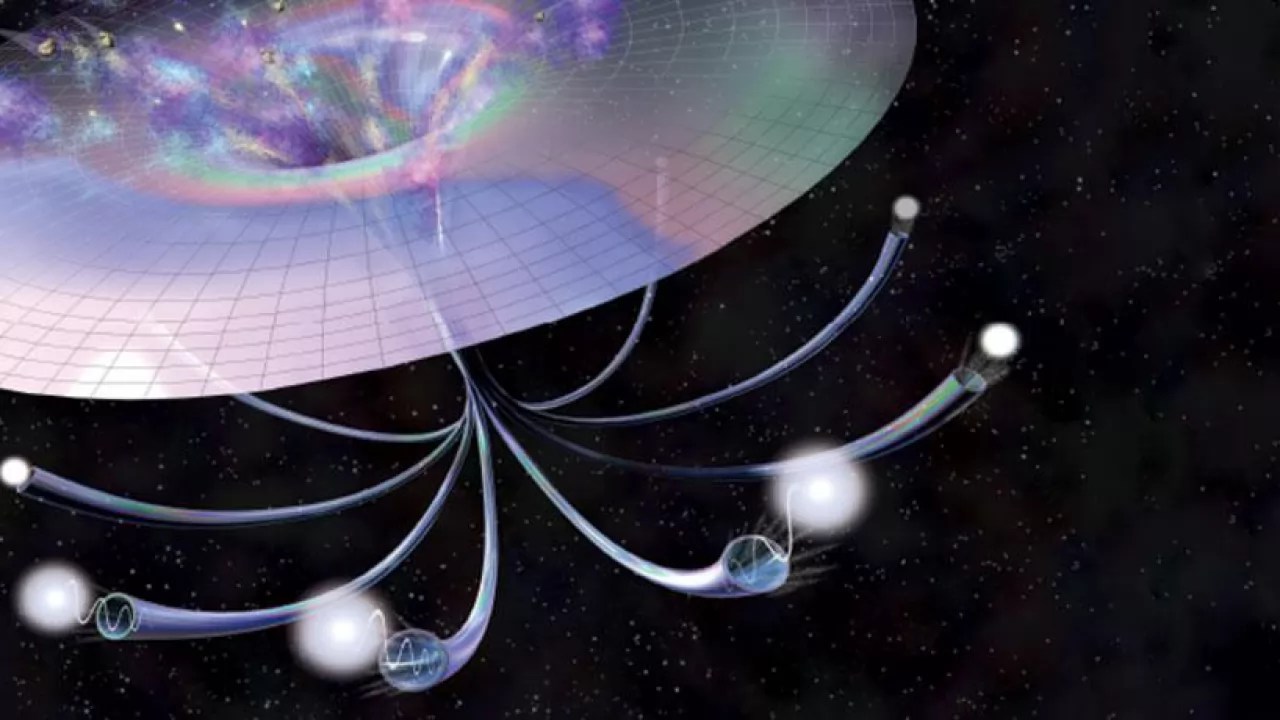
From whatever pre-existing state started it, inflation predicts that a series of independent universes will be spawned as inflation continues, with each one being completely disconnected from every other one, separated by more inflating space. One of these “bubbles,” where inflation ended, gave birth to our Universe some 13.8 billion years ago. Today, dark energy dominates the Universe and causes space to expand exponentially as well. These scenarios may be related, but we have no idea how long inflation persisted for prior to the hot Big Bang: only the ability to say, “at least 10^-32 seconds” or so.Credit: Nicolle Rager Fuller
However, we can state that the inflationary phase could only have lasted for a finite amount of time before giving rise to what we identify as our hot Big Bang, and that hot Big Bang occurred 13.8 billion years ago and has given rise to an observable Universe that is 46.1 billion light-years in radius, where the unobservable Universe is likely much larger.
If the Universe has always existed, if its birth occurred an infinite amount of time ago, or if it was born with infinite size, then the unobservable Universe must be infinite. If none of those things are true, then the Universe is forbidden to be infinite, and instead must be finite. As unfathomably large as an inflationary spacetime that gave rise to a multiverse of uncountably large numbers of hot Big Bangs may be, unless the Universe were born infinite in spatial extent or has existed for an infinite amount of time, it must still be finite in extent.
Regardless of whatever conditions existed that gave rise to inflation, and regardless of how long inflation endured for before our hot Big Bang began, those remain the only ways to have a physical Universe that’s infinite in spatial extent. Whether it’s finite or infinite, however, based on the evidence that’s even in principle observable to us, may be a question whose answer is fundamentally beyond science’s ability to answer.Share Ask Ethan: Is the Universe finite or infinite? on Facebook
Whenever we look out at the Universe, no matter how powerful our tools or how clever our techniques, there’s always a limit to what we’re capable of observing. No photons, or quanta of light, can be seen beyond the cosmic microwave background: a curtain of light that marks the first formation of stable, neutral atoms, occurring 380,000 years after the hot Big Bang. Prior to that, there is a neutrino signature — the cosmic neutrino background — that was emitted just 1 second after the hot Big Bang, and a theorized gravitational wave background, generated by the inflationary phase that set up and preceded the hot Big Bang, that is potentially detectable as well.
But all of these signals are fundamentally limited: by the amount of time they’ve been propagating and the fundamental limit at which an information-carrying signal can travel: the speed of light in a vacuum. That sets the ultimate limit of what’s in our observable Universe, and whatever part is unobservable, beyond that, can only be inferred, not directly measured. So what does that mean for the big question of whether the Universe, as a whole, is finite or infinite? That’s what our anonymous question-asker sent in this week, inquiring:
“With the observable universe so smaller than the real size of the universe, why do we assume the universe is finite rather than infinite in nature? With cosmic inflation as evidence, how do we assume it all started in a single place without variation from multiple places all at once?”
These are two big questions rolled into one, so let’s take them both on together!
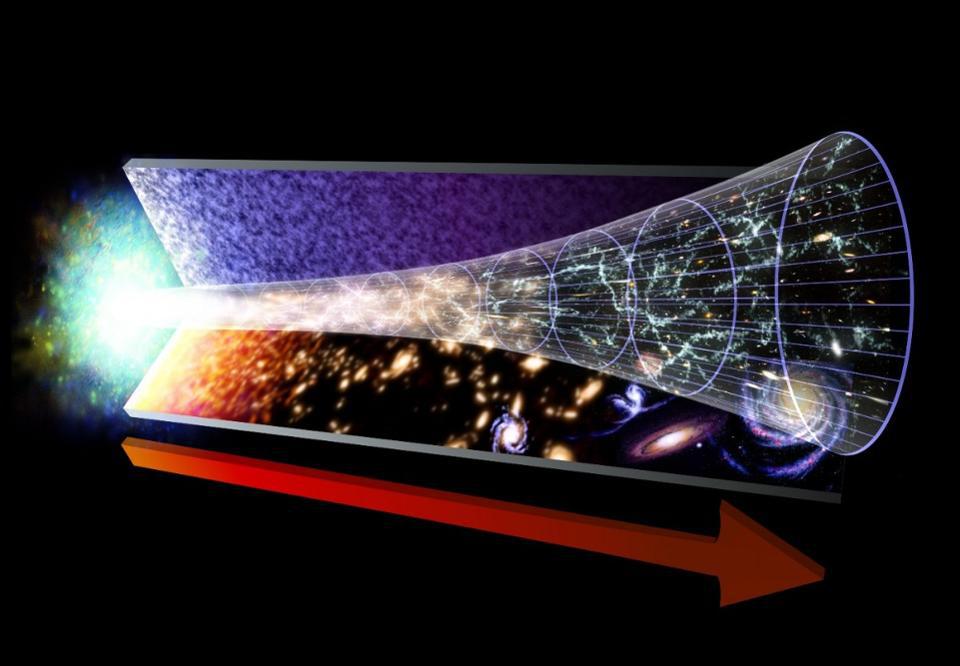
4There is a large suite of scientific evidence that supports the expanding Universe and the Big Bang. At every moment throughout our cosmic history for the first several billion years, the expansion rate and the total energy density balanced precisely, enabling our Universe to persist and form complex structures. This balance was essential if complex structures, like stars and galaxies, were to arise within the Universe.
Even though we only have access to the observable part of our Universe, there’s still a tremendous amount of information encoded within it. Just like a “footprint” can reveal all sorts of information about the animal that must have left it behind, the various processes that occurred during the first moments of the hot Big Bang (and even some moments that came before) leave imprints that persist even to the present day.
No comments:
Post a Comment